I’m currently on a 3-week trip to North America, and will be returning to Australia in mid-June. At the end of my travels I’ll be attending an energy futures conference in Waterloo, Canada (and will write more about that in an upcoming blog). But at present, I’m in Davis, California, and have been visiting Tom Blees. So it’s appropriate that I present a guest post from Tom, which was originally published in Meteorological Technology International magazine in May 2011. You can download the printable PDF version here.
Guest Post by Tom Blees. Tom an advanced energy systems consultant from Davis, California, and author of Prescription for the Planet – The Painless Remedy for Our Energy & Environmental Crises. Tom is also the president of the Science Council for Global Initiatives , an international think tank of distinguished scientists dedicated to creating an environmentally sound energy-rich future for the entire human race. Previous guest posts on BNC include: Unnatural Gas, Danish fairy tales – what can we learn? and Germany – crunched by the numbers.
The nuclear power plant debacle in Japan in the wake of the recent earthquake and tsunami has complicated what already was a contentious question: Should we look to nuclear power as a major component in solving the climate change problem? The situation at Fukushima Daiichi in Japan is getting more manageable by the day, though the ultimate repair and cleanup will be a long-term project. The 24-hour news cycle has feasted on the public’s dread of radiation, relegating the deaths of tens of thousands in the earthquake and tsunami to almost a footnote on American cable news shows. Anti-nuclear crusaders have been trotted out with little regard for their qualifications, some resurrecting long-debunked tales of deaths and injuries at Three Mile Island (where nobody was even hurt, much less killed).
The predicted nuclear renaissance may founder temporarily in some countries because of these events, but the lessons that will be learned from Japan’s accident won’t stop the growth of nuclear power in the long run. It will only make future plants safer. Despite the dire warnings of doomsayers, nuclear power plants being built today are far safer than those at Fukushima, and the Generation IV reactors to come will be even better. The aged power plants at Fukushima that would likely have survived the tsunami intact if not for the woefully misjudged placement of their backup power supplies had been running as long as forty years, and were designed half a century ago.
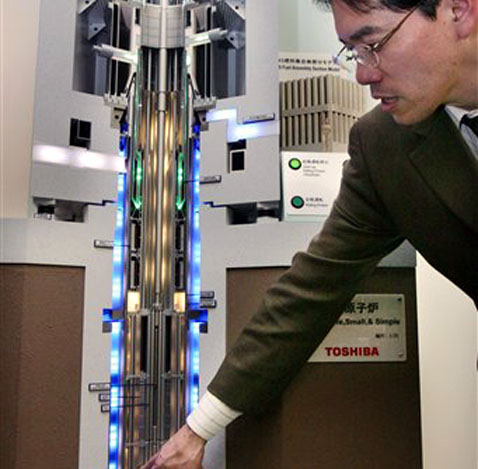
A Toshiba engineer describes features of the "4S" (super-safe, small and simple) nuclear battery – a sodium-cooled fast reactor with metal fuel, based on the IFR concept
Nuclear Technology Moves On
How’s that laptop working that your daddy bought you back in 1960? One might well pose that question to those who now advocate the wholesale abandonment of nuclear power based on the accident in Japan, for technology—nuclear and otherwise—has not been standing still. The fact is that our energy options are limited, and those that can provide baseload electricity (24/7 on demand) without carbon emissions are more limited still. Except for geothermal power opportunities accessible in just a few places in the world, hydroelectric power and nuclear power are just about the only two choices. Hydro, of course, while not as geographically limited as geothermal, nevertheless is circumscribed by both topography and politics. (On that latter point, it’s ironic that the Sierra Club used to be pro-nuclear until the early Seventies, seeing nuclear power as the way to obviate the building of dams. Since their complete reversal of that position they have been anti-nuclear crusaders—who still hate dams.)
Whatever one believes about the causes of climate change, there is no denying that glaciers around the world are receding at an alarming rate. Billions of people depend on such glaciers for their water supplies. We have already seen cases of civil strife and even warfare caused or exacerbated by competition over water supplies. Yet these are trifling spats when one considers that the approaching demographic avalanche will require us to supply about three billion more people with all the water they need within just four decades.
There is no avoiding the fact that the water for all these people—and even more, if the glaciers continue to recede, as expected—will have to come from the ocean. That means a deployment of desalination facilities on an almost unimaginable scale. Not only will it take staggering amounts of energy just to desalinate such a quantity, but moving the water to where it is needed will be an additional energy burden of prodigious proportions. Given the formidable energy requirements for these water demands alone—not to mention the energy demands of the developing countries for all their other needs—any illusions about wind turbines and solar panels being able to supply all the energy humanity requires should be put to rest.
Fortunately for all of us, the nuclear power technologies that can safely provide all the carbon-free energy that humanity will desire in the years to come have already been invented. We are already seeing the first of the so-called Generation III+ light-water reactors (LWRs) being built in China, the Westinghouse/Toshiba AP-1000. GE/Hitachi’s III+ design, the ESBWR (Economic Simplified Boiling Water Reactor), is slated to be certified for construction this fall by the U.S. Nuclear Regulatory Commission (NRC). Both reactors utilize advanced passive safety features that rely on the laws of physics rather than operator or automated intervention to deal with potential accident scenarios.
These reactors are also designed to weather electrical shutdowns like the one that bedeviled the Japanese plants and caused such a cascade of problems. With natural circulation only, the plants will remain in a safe condition even in an accident where no humans are on-site for three days. Probabilistic risk assessment studies for the ESBWR indicate that we could expect a core meltdown once every 29,000,000 years. But say we built 1000 of them (twice the total electrical generating capacity of the USA, and the amount of new nuclear the Chinese plan to build by 2050). That would mean you could expect a core meltdown once every 29,000 years, still virtually fail-safe. If we build ten times that many, 10,000 reactors, that would mean an expected core melt just once every 2,900 years, and as we can see from the experiences of Japan and Three Mile Island, you’d still probably have zero casualties, once every three millennia. Granting that even the most rigorous risk assessment studies might perhaps miss something, the safety margin is still so great that it would be the height of folly to abandon nuclear power when systems such as this are available to us. What would we use instead that can fill the bill?
Nuclear Waste and Material
But detractors will nevertheless complain that reactors like the ESBWR still produce long-lived radioactive waste products that will have to be safely watched over for what is, for all intents and purposes, forever (from a human standpoint). Another objection frequently raised is the risk of nuclear proliferation, the fear that nuclear material will be misdirected from power plants and made into nuclear weapons. Fuel supply is also an issue when the prospect of a burgeoning nuclear renaissance is considered, with demand for uranium expected to skyrocket. And over all this looms the capital cost of building nuclear power plants, which many consider a deal-breaker even if all the other issues could be resolved.
Back in the early Eighties a group of talented nuclear physicists and engineers realized that if there was to be any reasonable expectation of widespread public acceptance of nuclear power, all these problems would have to be solved. So they set out to solve them. Under the leadership of Dr. Charles Till at Argonne National Laboratory’s western branch in the state of Idaho, a virtual army of nuclear professionals designed an energy system that many expect will soon power the planet, if only we can muster the political will to deploy it. Their test reactor operated virtually flawlessly for thirty years as they identified and solved one potential obstacle after another, proceeding methodically until they were ready to demonstrate the commercial-scale viability of their revolutionary fuel recycling system that would complete what had been a spectacularly successful project. What they had accomplished during those years was, without exaggeration, probably the most important energy system ever invented, one that promises virtually unlimited safe, clean energy for the entire planet. Unfortunately, an almost unbelievable shortsightedness on the part of politicians in Washington D.C. pulled the plug on the project just as it reached its final stage in 1994, and the promise of the Integral Fast Reactor (IFR) languished virtually unnoticed for the next fifteen years.
The Integral Fast Reactor
But the IFR is such a grand invention that it couldn’t stay buried any longer, and people around the world are now clamoring for it to be deployed. The looming threat of climate change has prompted many to take a fresh look at nuclear power. Some have considered the problem of so-called “nuclear waste” (not waste at all, as we shall soon see) an acceptable price to pay in order to curtail greenhouse gas emissions. In the wake of the Japan accident, safety will also be prominent in the debate. The IFR, though, is so impressive in its qualifications that even previously hard-core anti-nuclear activists have touted it as the ultimate answer. And the fact that over 300 reactor-years of experience have been accumulated with fast reactors around the world means that such technology is no pipe dream, but a mature technology ripe for commercial deployment.
The term Integral Fast Reactor denotes two distinct parts: A sodium-cooled fast neutron fission reactor and a recycling facility to process the spent fuel. A single recycling facility would be co-located with a cluster of reactors. Figure 1 shows a simplified version of such a reactor. It consists of a stainless steel tub of sodium, a metal that liquifies at about the boiling point of water. Sodium is used both as a completely non-corrosive coolant and, in a separate non-radioactive loop, as the heat transfer agent to transport the heat to a steam generator in a separate structure (thus avoiding any possible sodium-water interaction in the reactor structure).
The system is unpressurized, and the pumps are electromagnetic pumps with no moving parts. In the event of a loss of flow, natural convection and the large amount of sodium will be sufficient to dissipate the heat from the fission products in the core, unlike the situation in the Japanese reactors at Fukushima, which required constant cooling even though the reactors had been shut off.
The commercial-scale iteration of the IFR’s reactor component is called the PRISM (or its slightly larger successor, the S-PRISM, though for the sake of brevity I’ll hereafter call it simply the PRISM, which stands for Power Reactor Innovative Small Module). It was designed by a consortium of American companies in conjunction with Argonne Lab, and is now being further refined by GE/Hitachi Nuclear. From a safety standpoint it is unparalleled. If the risk assessment studies for the ESBWR mentioned above sound impressive, those of the IFR are even better.
In my book Prescription for the Planet, I did a thought experiment based on the risk assessment studies for the PRISM that have already gotten a preliminary nod from the NRC. The likelihood of a core meltdown was so improbable that I figured out how often we could expect one if thousands of PRISMs were providing all the energy (not just electricity) that humanity will require a few decades hence (according to most estimates). Remember, the occurrence of one meltdown would require dividing the total number of reactors into the probability for a single reactor. Even so, the probable core meltdown frequency came to once every 435,000 years! Even if that risk assessment was exaggerated by ten thousand times, it would still mean we could expect a meltdown about once every half-century for all the energy humanity needs.
Reactors and Natural Disasters
The crisis at Fukushima’s power plant has stoked fears that existing nuclear sites may be incapable of withstanding quakes in excess of their design specifications. Whereas many lightwater reactors are designed to withstand G forces of about 0.3, the PRISM is rated at 1.0. This G rating is different than a Richter scale rating because the Richter scale represents the total energy released in an earthquake, which is dependent on many factors (duration, depth, etc.). When designing a structure or piece of equipment to withstand earthquakes, the degree of ground acceleration is what matters. If one were to stand directly on a geological fault line during the most severe earthquake imaginable, the G forces caused by ground acceleration would almost certainly not exceed 1.0. (The maximum ground motion at the Fukushima complex during the earthquake measuring 9.0 on the Richter scale was 0.56 G) So the PRISM reactor, designed for that level of motion, could safely be built in any seismically active area. Of course it goes without saying that no power plant should be built at a low elevation in a zone that is vulnerable to tsunamis, or for that matter on a flood plain. But with the PRISM, seismic shocks are not an issue.
As for proliferation risk, it should be pointed out that the risk of proliferation from any sort of power reactor has been substantially mischaracterized and generally overblown. The reason is that the isotopic composition of the uranium and plutonium in power reactors is lousy for making weapons. Any country that wishes to pursue a weapons program covertly is far better served by using a small research reactor operated in a specific manner to produce high-grade weapons material, and even then it requires a quite complex reprocessing system to separate it.
That being said, the IFR system uses a unique metal fuel that can not only be easily and cheaply recycled on-site and then fabricated into new fuel elements, but at no stage of the fuel cycle is any sort of weapons-grade material isolated. All the isotopes of uranium and plutonium are not only left mixed with their various cousins, but there is always at least a bit of highly radioactive fission product elements, making the fuel impossible to handle except by remote systems.

Figure 2: The fission products will only be radioactive beyond the level of natural ore for a few hundred years.
The buildup of such fission products in the fuel, though, is what eventually necessitates pulling fuel elements out of the reactor for recycling. In the pyroprocessing system—a type of electrorefining common in the metallurgical industry but unique to the IFR among reactor systems—the majority of the fission products are isolated. The rest of the fuel is reincorporated into new fuel elements. The fission products, representing only a small percentage of the fuel, are entombed in borosilicate glass that can’t leach any of them into the environment for thousands of years. Yet the fission products will only be radioactive beyond the level of natural ore for a few hundred years (see Figure 2). Thus the so-called “million year waste problem” is neatly solved.
As for the question of uranium supply, that issue is moot once we begin to build IFRs. First we’ll use up all the spent fuel that’s been generated over the years by LWRs, plus all the weapons-grade uranium and plutonium from decommissioned nuclear weapons. It’s all perfect for fuel in IFRs. But then when that’s all gone we can fuel them with depleted uranium. There is already so much of it out of the ground from years of nuclear power use that even if we were to supply all the energy humanity is likely to need from just IFRs alone, we’ve got enough fuel already at hand for nearly a thousand years. As efficient as LWRs are in squeezing a huge amount of energy out of a small amount of fuel, fast reactors like the PRISM are about 150 times more efficient. In fact, all the energy a profligate American would be likely to use in a lifetime could be extracted from a piece of depleted uranium the size of half a ping-pong ball.
Finally we come to the clincher: the cost. For some reason it supposedly is going to cost anywhere from two to five times as much to build a nuclear power plant in the USA than exactly the same design being built in the Far East. This comparison applies not just to countries with low labor costs but to Japan too, where labor costs are high and nearly all the materials are imported. It’s an American societal and political problem, not an inherent flaw of nuclear power. Utility companies fear that a group of protesters with signs and lawyers might shut down construction midway through a multi-billion-dollar project, or prevent a built reactor from operating. So they prudently try to build that uncertainty into their cost estimates (with maybe a little padding to boot).
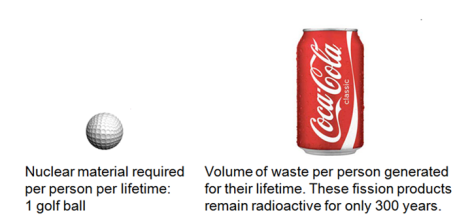
A golf ball of uranium would provide more than enough energy for your entire lifetime, including electricity for homes, vehicles and mobile devices, synthetic fuels for vehicles (including tractors to produce your food and jet fuel for your flights). Your legacy? A soda can of fission product was, that would be less radioactive than natural uranium ore in 300 years.
The new reactor designs, both the Gen III+ designs mentioned earlier and the PRISM, are designed to be mass-produced in modules, then assembled at the power plant site. The PRISM has the added advantage of operating at atmospheric pressure, so no pressure vessel or high-pressure pumps are needed. The passive safety principles mean that multiple redundancy is unnecessary, allowing such reactors to have far fewer pumps, valves, controls, and other components than their older Gen II predecessors. Based on both industry estimates and actual experience of building these reactors since the Nineties, there is every reason to believe that the price can be kept well below $2,000/kW, though the Chinese plan to produce them for half that price once their mass production supply lines are in place.
There is virtually no doubt that with these new nuclear technologies available, the shift to predominantly nuclear power is virtually inevitable in the long term. Over sixty new plants are under construction around the world with many more to come, even if some nations are temporarily deterred by political and social pressures. If we’re serious about solving the climate change problem before it’s too late, we’ll have to get serious about the only zero-emission baseload power source that can easily supply all the energy the world needs.
We shouldn’t consider this a Faustian bargain. These new designs—particularly the IFR—are clean, safe, economical, and able to convert waste products that we desperately want to get rid of into abundant energy for the entire planet. Anyone serious about protecting the environment can safely embrace them with enthusiasm.
Following.
following
With the eventual exception of fuel supply, all of nuclear energies problems are political, and ether fabrications, or the consequences of fabrications. While Fast Reactors, must be the future, I again caution those who would dwell too much on the point that these can solve these fabricated issues.
The problem is that those that made up these lies in the past are quite capable of doing so again, and there are aspects of these Gen III+ systems that can be spun, and misinterpreted as was the case with their predecessors.
For example the ‘feature’ of spent fast reactor fuel that it is too hot to handle, and therefore proliferation resistant, will be spun into statements that these reactors are bad because the spent fuel is more radioactive. Yes it isn’t going to be for as long, but that isn’t the story the anti’s will tell.
Nor will they stop there. The point is that these reactors can and should stand on their, more pertinent advantages, and pandering to these other artificial issues should be avoided.
In the Wikipedia page about the Christchurch earthquake, ( http://en.wikipedia.org/wiki/2011_Christchurch_earthquake ) you can see that the maximum acceleration went up to 2.2g, with 1.8g in the city centre. You wouldn’t have wanted a PRISM reactor there…
Note that it was only from an earthquake of magnitude 6.3 (actually only an aftershock of the Canterbury earthquake) and, more importantly, from a fault line that was not previously known ! From a nuclear siting standpoint, this earthquake was even more troubling than the Fukushima earthquake.
After these two, I tend to put my faith more into floating or submerged NPP, which could perfectly be of PRISM design.
I think that an estimate of a core meltdown every 29,000,000 years is limited. How can it take into account the risk that over that time some individual or group will sabotage or bomb a reactor, especially if the risk is spread over many reactors in many different nations.
I don’t think any discussion of nuclear power is complete without talking about the human factors of war and terrorism, even if the proliferation issue can be seen to be separate. How much worse would the situation in Fukushima be if the damage had been caused by humans placing a large shape charge against the pressure vessel, or dropping a large conventional bomb on it?
Since these issues are absent from the pro-nuclear articles, I can only assume there isn’t a good answer.
Of course, this doesn’t mean that wind, solar, etc. are the answer either.
@Tom – as DV82XL points out, many of the objections to nuclear fission energy are merely fabrications – aka fictions – created by people for whom the following very hopeful and comforting passage is scary as heck:
“What they had accomplished during those years was, without exaggeration, probably the most important energy system ever invented, one that promises virtually unlimited safe, clean energy for the entire planet.”
Suppose that you are sitting on top of the world’s economy, but your position is based on having control of traditional energy supplies. How would you feel if someone actually succeeded in producing unlimited, safe, clean energy and was willing to share that with the entire planet? Where would you market your dirty, expensive hydrocarbons – the Moon or Mars?
The Administration that was in office in 1994 was keenly aware that hydrocarbons do not have inherent value – they have value when they are the best available energy choice. That value is enhanced when people know that the rate of production is limited and that there is competition for access to the supply. That helps encourage the highly profitable market bidding war that some call “speculation”.
“Unfortunately, an almost unbelievable shortsightedness on the part of politicians in Washington D.C. pulled the plug on the project just as it reached its final stage in 1994, and the promise of the Integral Fast Reactor (IFR) languished virtually unnoticed for the next fifteen years.”
Imagine for a moment that the Secretary of Energy and the Chief of Staff were both former natural gas executives and that the President was a formerly poor man who used his personal charm to build a profitable political career in one of the few states in the country that actually produces more fuel than it uses. Then imagine that one of the most powerful leaders in the Senate was a politician from a state that has been dependent upon income from coal, oil and gas for its 125 year history of existence.
The IFR was put on the shelf by people with a long term outlook, but one that was entirely focused on their own profits, not on the good of humanity.
In my view, that is the primary source of the strength of the anti-nuclear movement around the world – it has the strong support of the fossil fuel establishment.
Hey Tom: do you have a source for the Chinese plan to produce newer reactors for ~ 1000/kw?
From what you wrote above, it appears that “these reactors” refers both to Gen Three and PRISMs.
when you refer to “actual experience of building these reactors,” are you referring here to gen three, four or both?
following
Good post; a detail here:
You write:
> the dire warnings of Cassandras
Wrong word, wrong fable, wrong concept:
http://www.pantheon.org/articles/c/cassandra.html
Cassandra got it _right_ repeatedly, never believed. That’s not what you’re describing, not at all.
You are mixing several _other_ concepts there:
— people who “cry wolf” — “alarmists” — liars who, by raising false alarms repeatedly, lose credibility, so later their real warnings get ignored
http://en.wikipedia.org/wiki/The_Boy_Who_Cried_Wolf
“‘… when we are alarmed with imaginary dangers in respect of the public, till the cry grows quite stale and threadbare, how can it be expected we should know when to guard ourselves against real ones?’[6]
-or-
— people who “poison the well” — lying about _others_ to undercut their legitimate warnings
http://en.wikipedia.org/wiki/Poisoning_the_well
“… A subcategory … is the application of an unfavorable attribute to any future opponents, in an attempt to discourage debate.” We get that a lot.
——
For the Chinese, the “cry wolf” story is told about “King You of Zhou” — same idea:
http://en.wikipedia.org/wiki/King_You_of_Zhou
_____________________
———————————-
Nitpicking: a basis of the primate social bond.
Nitpick a friend today; it’s good for everyone.
@Gregory:
http://www.world-nuclear.org/info/inf63.html
This is the Chinese nuclear information page that is update more or less very month.
The Chinese are building their version of a PBMR called HTR. They are *about* to break ground on this 160MW reactor but are holding it up because of Fukushima. The’ve been running a 10MW R&D version for a few years. Walk away safe: they pulled all power to it and sat their for hours watching the heat dissipate.
LFTR. In February the Chinese announced support for deployment of their version of a “Thorium Molten Salt Reactor”. Likely this will be in the sub-500MW range.
Put advanced HEPA and activated carbon filters on passive emergency vent lines and you’ve protected the public even if you have a core meltdown a la Fukushima Daiichi:
http://www.emcelfilters.co.uk/nuclear_filters/cylindrical_hepa_filter2.htm
http://www.emcelfilters.co.uk/nuclear_filters/divided_bed.htm
No valves or anything required.
Hmm, 99.999995 percent efficient nuclear particulate filters:
http://en.wikipedia.org/wiki/HEPA
Another nitpick: you write
” … to withstand earthquakes, the degree of ground acceleration is what matters.”
I realize you’re trying to simplify but that’s outdated. It oversimplifies what we have learned since the 1980s, from actual experience rather badly.
See, e.g.:
http://nisee.berkeley.edu/lessons/singh.html
“to select a design earthquake …. requires a good understanding of the ground motion parameters that characterize the severity and the damage potential of the earthquake ….
Early reliance on peak ground acceleration seemed very natural to engineers since Newton’s Second Law clearly sets acceleration and the resulting inertial forces in direct proportion. However, more often than not, the peak acceleration corresponds to high frequencies which are out of the range of the natural frequencies of most structures. ….
…. peak ground acceleration is the parameter most often associated with severity of ground motion. However, it has generally come to be recognized that this is a poor parameter for evaluating the damage potential…..”
> so-called “nuclear waste” (not waste at all, …
Yes — DONE — you can cite Stephen Chu (2009)
“Steven Chu: Yucca Mountain as a repository is off the table…. we know a lot more today than we did 25 or 30 years ago…. dry cask storage at current sites would be safe for many decades, so that gives us time to figure out … a long-term strategy….
[We’re] looking at reactors that have a high-energy neutron spectrum that can actually allow you to burn down the long-lived actinide waste….”
http://www.businessinsider.com/steven-chu-on-solving-the-nuclear-waste-problem-2009-5
quoting from a MIT Technology Review interview at
http://www.technologyreview.com/business/22651/
See also: http://www.technologyreview.com/blog/energy/26744/ which mentions a variety of things
-fusion designs continue to evolve
http://www.generalfusion.com/generator_design.html
and
-smart-grid operation tested in Ohio
http://news.duke-energy.com/2011/05/04/self-healing-operation/
To reinforce Tom’s point that reactor safety has improved since the Fukushima Daiichi reactors were built, consider the sister station, Fukushima Daini. Four reactors there, but with post-1980 designs. They were hit by the same tsunami — but there were no explosions and no radiation releases, so we don’t hear about them.
Even late Gen-II reactors remained contained.
See http://snipurl.com/27xo8g.
Even if we want to eventually store spent fuel in geologic storage, it makes sense to store it in dry casks for at least a century due to reduced heat load in the repository (you can put more spent fuel in) because heat load is the limiting factor for a repository. Of course it is my opinion that putting stuff underground is a bad idea in the first place, at best a waste of precious financial and intellectual resources. Much better idea is to spend the money in advanced pyro processing techniques. Lets put a deadline in for the sceptics: give us 50 years to develop the reprocessing technologies, if it works we have only short term (fission product) storage, if it doesn’t work we have much cooled down spent nuclear fuel that is easier to store permanently in the repository. Either way is a big improvement over todays crazy flip flopping politicians lack of vision.
Fusion designs continue to evolve, nonsense fusion has never generated a kWh of electricity and this is no different today than 50 years ago.
Fusion is very difficult. Neutronic fusion means 14 MeV neutrons ripping apart all materials even theoretically conceivable, whereas aneutronic fusion has too high threshold breakeven practicalities.
Fission is very simple. It wants to fission. It is heavy stuff. Very unhappy being heavy, they want to fission. Chain reaction easy to sustain. Done effectively and affordable for over half a century. World war II technology. Proven, just what we need.
We don’t need anymore pies in the sky. We’re starving and the pies in the sky are distracting use from using the food we know we can get.
Fossil fuels kill indiscriminantly. Radiophobia still grips societies throats. Solar and wind don’t work because they’re not there 70-95% of the time.
So its more fossil. Get used to it. Fossil fuels are here to stay.
What about the sodium coolant? Seems like a serious drawback of the IFR technology compared to other fast reactors or thermal breeders like the MSR. Why not use lead or helium as the coolant for your reactor? Both options are safer, and permit higher outlet temperatures, allowing for the thermochemical production of hydrogen in addition to electric power. As far as I know the Russians have plenty experience in the operation of lead-cooled fast reactors.
> it makes sense to store it in dry casks …
> … putting stuff underground is a bad idea
Exactly; and that’s current policy, see the Stephen Chu interview linked above.
You got ‘yes’ for an answer, you can stop arguing.
Look at the ‘blue-ribbon panel’ membership, do you know the current plans now being discussed?
Tali, on 28 May 2011 at 11:05 PM said:
I don’t think any discussion of nuclear power is complete without talking about the human factors of war and terrorism, even if the proliferation issue can be seen to be separate.
The human factors of war and terrorism are that there is an almost uncountable number of ‘large soft targets’. Poison gas in a subway system, poisoning the water supply, crashing a jet into a sports stadium, derailing a passenger train etc etc etc… Targets tend to be chosen because they have military or political significance or because they are ‘easy’.
Tom: Your statement: “there is no denying that glaciers around the world are receding at an alarming rate. Billions of people depend on such glaciers for their water supplies.” is not true.
Galciers are snow that hasn’t melted. They are ice. They are not drinking water supplies.
People drink water from rivers that come from rain, monsoon rains and from melted snow. People dont drink glaciers, at least intact ones. Melting glaciers may supply a tiny amount of water yes, but intact glaciers no.
And yet no sodium-cooled reactor has ever harmed anyone.
Fuel can overheat, and leak into the surrounding sodium, but then, due to the great stability of sodium iodide, that is as far as the iodine goes.
Except in the case of metallic fuel; then, the great stability of uranium iodide means the iodine-131 doesn’t even go that far.
Forgot to link: in re my 3:53 AM post: http://www.etec.energy.gov/library/SRE_Accident/Christian_Report_on_SRE.pdf . It discusses historical instances of fuel overheating in sodium.
Helium is high pressure which has its own class of safety problems (as well as economics problems).
Lead is corrosive to most metals (it forms solutions with them) whereas sodium doesn’t.
But sodium is reactive. And like lead it is intransparent and has a poor volumetric heat capacity.
I prefer fluoride as a coolant. NaF-BeF2 eutectic melts at 340 degrees Celcius, acceptable. Very high heat capacity, about 4x higher than sodium per volume, and it is transparent. So you can view status of the core and pool much easier. Plus it doesn’t react violently with water/air unlike sodium. It has a boiling point in excess of 1300 degrees Celcius so it can be used for very high temperatures (but also at the standard sodium IFR temperatures).
Iodine in a metal fuelled reactor will not only bond to uranium but also to the fission products, in particular cesium and strontium. So that helps take care of those two buggers as well.
Iodine will also dissolve in fluoride salts; it’s not going anywhere. The filtered confinement containment will use HEPA and carbon filters to remove any traces of these elements during a severe accident (extremely unlikely for this reactor type due to passive decay heat cooling).
@harrywr2
“The human factors of war and terrorism are that there is an almost uncountable number of ‘large soft targets’. Poison gas in a subway system, poisoning the water supply, crashing a jet into a sports stadium, derailing a passenger train etc etc etc… Targets tend to be chosen because they have military or political significance or because they are ‘easy’.”
So, basically, you’re saying we should not worry about what might happen to an LFTR, ESBWR or PRISM, etc., reactor in the event of an act of sabotage or war because there are a bunch of other things that people will blow up first because there easier or will kill more? How can you suggest that these reactors won’t have “military or political” significance when we’re talking about using them to replace the current role played by oil, coal and gas?
But, we don’t know how hard it would be to attack a reactor, nor how much death would result, because it isn’t discussed in the article. The 2,900 years between meltdowns for 10,000 ESBWR reactors isn’t credible because some of those reactors will likely end up being built in states that will later fail, etc., or be become the target of a bunch of millenarian cultists with guns and money. Such scenarios are likely to bring about much more than a core meltdown.
How bad would it be?
> People dont drink glaciers
This is silly argument.
Glaciers, and snowpack, dependably moderate precipitation runoff. That’s one way we depend on them as a water supply — we depend on them existing-being there-over the long term.
@ Tali 0n 29 May 2011 at 4:15 AM.
Life has two major aspects.First,it is a continual process of solving problems.Second,life is full of risk.
In the course of solving a problem there are risks to be taken.A prudent person does not refuse to tackle the problem because of risks.He/she endeavours to reduce the risks to a reasonable level of probability.
Terrorism is a border control,intelligence and policing issue no matter what the target of the terrorists is.A nuclear reactor of any sort with normal security applied would be a much harder target than a bus or train full of people.Do you avoid all public places with large numbers of people present?
As for war,the targets will vary according to the strategy of the parties.Any electricity supply facility could be a legitimate target.If occupation of the disputed territory is an object then blowing up a nuclear reactor would not be very sensible.
The fact is,Tali,your harping on the war/terrorism aspect of nuclear is just a syptom of your anti-nuclear mindset which,I put it to you,is irrational at best.
It is well past time for you and your ilk to stand back and consider what effect you are having on the ability of rational people to solve some of the massive problems the world has.
Apply the same argument to other technologies and you see that there’s no point.
Aircraft: lets not build aircraft because some terrorist group might use it to kill thousands.
Skyscrapers: lets not build skyscrapers because the above people might use their aircraft to fly into it.
For some reason people still use this argument for not building nuclear power plants all around the world. Its silly and not grounded in reality.
Modern life requires dealing with risks and making tough choices. Fossil fuels are far more dangerous than nuclear plants. They routinely kill millions while anti-nukes worry about hypothetical scenarios that kill thousands.
We can make simple passive adjustments to nuclear plants, such as turbine driven pumps with electrical generators attached, diesel generators higher up in shielded bunkers to prevent flooding and guard against bombs, tornadoes and such, advanced filter systems that are passively actuated by rupture discs to vent the steam while grabbing 99.99 percent of the radioactivity when the containment design pressure is exceeded, etc. Many of these are practical and cheap solutions. Constrast this to removing 99.99 percent of the particulate matter and 99% of the CO2 for a coal plant – technologically challenging due to the large mass flows, and therefore not economical. A large coal plant produces a ton of CO2 every 4 seconds! You don’t just sweep that under the carpet. Storing this stuff itself is a major hazard; what if the hundred million tonnes of CO2 produced finds its way and kills thousands?
And what if a freak solar low period causes all solar panels in the world to produce very little power, plunging the world into a medieval setting, with murdering and raping millions as a consequence?
By the way, while we were discussing theoretical situations like that, a couple of thousand people died prematurely due to fossil fuel combustion.
i also disagree with several points in the article.
we will have to wait until we get the full data, but if the Fukushima reactors have been damaged by the earthquake, we need reactors that will work while the coolant is leaking out.
i fear this is difficult for all designs.
on the near future of nuclear power, it is interesting to look at the chart of plants near complete construction: (table at the bottom, i think some dates have been delayed already)
http://www.world-nuclear.org/info/inf17.html
i count 13 plants finished in 2011, but with Germany switching of 7 and Japan 6, we seem to face a year with zero growth.
This reference discusses the trapping of volatilized cesium:
http://www.osti.gov/bridge/servlets/purl/14899-R29lfC/14899.pdf
It appears a 99.99% removal efficiency is readily attainable, consistent with nuclear filter supplier claims. Using more carbon it might be possible to get 99.999% in a practical system.
Put such a system on your emergency venting line (and replace the valve with a rupture disc) and everyone’s safe even in a severe meltdown.
@G.R.L. Cowan:
I know of two fatal SFR accidents:
* US experimental reactor SL-1, which had a criticality accident killing 3; [1]
* French experimental reactor ‘Rapsodie’ at Cadarache, which had a sodium explosion during decommissioning, injuring 4 and killing 1; [2,3]
[1] http://en.wikipedia.org/wiki/SL-1
[2] http://www.nytimes.com/1994/04/01/world/reactor-explodes-in-france-killing-1.html
[3] http://www.iaea.org/inis/collection/NCLCollectionStore/_Public/31/040/31040829.pdf
Hank – if it’s silly, name some cities that get a substantial amount of their water from glaciers. Tom Blees thinks that “billions of people depend on such glaciers for their water supples.” Name a few.
> two fatal SFR accidents
Yep. Easy to find. Important to get this right.
> removal efficiency
> rupture disk
Cyril, these details are analyzed extensively in the docs from when the hardened vent system was proposed. You’re saying they did it wrong; you might look at the record as to why they did what they decided.
Here’s the Japanese industry’s response to TMI — showing the addition of both a hardened vent and a rupture disk:
http://sacre.web.psi.ch/ISAMM2009/oecd-sami2001/Presentations/p6-M.Kajimoto-BWR/SAMBWR.pdf
“Evaluation of Technological Appropriateness of the Implemented Accident Management Measures
for BWR …”
“The results indicated that accident management countermeasures implemented to BWRs in Japan were effective ….”
Other docs report that industry decided not to use a rupture disk because they wanted to be able to turn the emergency vent system _off_ using a valve.
@Podargus: I’m not really anti-nuclear, so you can hold back on the invective. My reaction was against Tom Blees’ quoting the 29,000,000 years between meltdown figure since I think it is a meaningless statistic in the face of real world human factors.
@Cyril R:
“Aircraft: lets not build aircraft because some terrorist group might use it to kill thousands.
Skyscrapers: lets not build skyscrapers because the above people might use their aircraft to fly into it.”
I was in lower Manhattan in August 2008. You’d never know what happened. The place was vibrant and bustling. Could we expect the same in an area around a nuclear reactor after a major sabotage or bombing?
So my chances of being affected by a terrorist attack on a reactor are negligible compared to other risks, but the chances of it happening somewhere seem much higher than 1 in 29,000,000 years of reactor operating time. So, let’s lay off the stupid statistics and be open about the fact that the worst case scenario for reactors is sabotage and the like. Glossing over it is pointless, because the opponents of nuclear power aren’t going to ignore it.
@Hank Roberts – Having lost any real arguments here, have you now been reduced to flipping firecrackers into people’s laps?
I don’t see anything in this thread from you other than sniping for its own sake. You’re not making any point other than the nuclear industry isn’t perfect, something no one here has ever asserted.
It’s a stale tactic, used by the antinuclear side for years, trying to put nuclear on a pedestal for the purpose of knocking it down.
When other major forms of generation, and I don’t mean pissant excuses for it like wind and solar. come close to nuclear’s record for safety, and environmental footprint, we can argue fine differences, before that time, it is an open attempt to muddy the waters.
uvdiv, SL-1 was a water-cooled reactor (see p11). And while the Rapsodie reactor was sodium cooled, and the accident was intrinsically associated with sodium clean-up, it was also 10 years after shutdown. So there is some leeway here for missing the second, and no reason for citing the first.
Tom Blees,
Thank you for a very helpful post full of interesting information about nuclear reactors.
The only thing that struck a jarring note was your comment on glaciers. Sure there are some negative consequences as a result of the continued melting of glaciers but these are insignificant in comparison with the benefits.
Only 20,000 years ago the Laurentide glacier covered most of Canada and over a quarter of the USA; it extended a mile deep to where New York City is today.
Farmers in Canada and America’s heartland are shedding no tears for the departed glacier.
Tom Blees,
Excellent post. Thank you
“ It’s an American societal and political problem. It’s an even bigger societal and political problem in Australia. So much so that nuclear is banned in Australia.
Re: proliferation/terrrorists
Tom Blees wrote:
“That being said, the IFR system uses a unique metal fuel that can not only be easily and cheaply recycled on-site and then fabricated into new fuel elements, but at no stage of the fuel cycle is any sort of weapons-grade material isolated. All the isotopes of uranium and plutonium are not only left mixed with their various cousins, but there is always at least a bit of highly radioactive fission product elements, making the fuel impossible to handle except by remote systems”
Umm. You’re not thinking out of the box. The fuel is impossible to handle SAFELY without remote systems. If you have characters prepared to die for the cause they could metaphorically, almost literally, handle it with their bare hands and not bat an eyelid.
IFRs have been tried and abandoned, superphenix being the most notable failure, the constant sodium fires made it intermittent, unreliable, expensive, and dangerous.
http://en.wikipedia.org/wiki/Superphénix
Really the worst of all worlds. And it STILL does not solve the problem of fission product nuclear waste, it makes it less per kWh though.
“When other major forms of generation, and I don’t mean pissant excuses for it like wind and solar.”
You can dismiss it all you want but this is reality
http://www.thegreenpages.com.au/news/sustainability-world-first-report-on-new-energy-capacity-shows-renewable-outweighs-nuclear-post-fukushima/
Yes I know just nameplate capacity, but with Nuclear slowing down after Fuskushima, and renewables rising, its only a matter of time before 50% of all new powerplants per kWh of yearly energy is renewables. It
Its not just a toy technology for hippies, but the inevitable future.
>For Leo Hansen:
http://www.sciencemag.org/content/328/5984/1382.short
“… meltwater is extremely important in the Indus basin and important for the Brahmaputra basin …. The Brahmaputra and Indus basins are most susceptible to reductions of flow, threatening the food security of an estimated 60 million people.”
> having lost any real arguments here
That’s why I’m here, to acquire convincing facts I can show others. I can’t just say “that guy is really sure” — I need to go on by saying “and he can show you facts, and I looked up what he said and he’s consistently got the facts right.”
Be convincing.
Yucca Mountain and deep burial, the old Reagan-era plans, didn’t work out. Obama’s administration is pushing dry cask storage and Gen4 reactors to burn transuranics, because climate change is such a concern. The industry didn’t implement the suggested burst disk design when adding hardened vents, preferring valves and fans.
Nothing to argue about there, once you look up the facts; those aren’t matters of opinion once cited.
What’s to argue about?
Climate change and health. How? Not by contradicting people — by showing facts.
“Argument is an intellectual process. Contradiction is just the automatic gainsaying of any statement the other person makes.”
http://www.mtholyoke.edu/~ebarnes/python/argument-clinic.htm
Climate change shows up in Antarctica:
-Progressive increase in ice discharge from fastest Greenland/Antarctic glaciers…. Likelihood of continued, very large increases in ice discharge
http://www.agu.org/pubs/crossref/2011/2011GL047304.shtml
Air pollution affects healthy people, not just sick people who could move away from the problem:
Time scale effects in acute association between air-pollution and mortality
-Strong and non-linear timescale effects between air-pollution and mortality …. Evidence of acute air-pollution health effects at the general population
http://www.agu.org/pubs/crossref/2011/2011GL046872.shtml
Useful facts. Great need to be convincing about the overall issue and the reasons for the need.
Hank: ” meltwater is extremely important” Please come up with a reference that says “glacier meltwater”. We all know that snow melts and provides river water. And 60 million people are a far cry from billions. (personal comment deleted)
@ Tali, on 28 May 2011 at 11:05 PM and again on 29th May:
I don’t know what kind of answer Tali would find adequate. So far, several answers have centred on softer targets and the fact that risks come in all shapes and sizes.
Terrorist acts against nuclear power stations are conceivable but not credible, simply because there are simpler options available for those who would specifically choose to render NPP’s ineffective.
My example is the island of Bougainville, off the eastern end of Papua New Guinea. Pro-independence activists were determined to shut down the major industry on the island, Bougainville Copper, which was a huge pit. The power station was at the coast, the mine was in the hills.
Rather than try to disable the power station, the insurgents took out the transmission lines and switchyards, over and over again. Their campaign to close the mine was successful. Decades later, long after hostilities ceased, the mine has not re-opened.
This demonstrates a universal truth: Power stations have weak links, but the power distribution systems are weaker and are thus more attractive to terrorists.
Tali should be more concerned about addressing the possible causes of social instability and potential war. The major threat right now seems to be GHG/climate change caused hardship for millions. As I see it, the best possible single response to this threat is fission power, and lots of it, on every continent of the globe.
So, Tali would be wise to use his effort to promote fission energy rather than to promote scare campaigns based on muddled thinking about an imagined, but never observed, threat due to terrorism.
Dealing wth the real and escalating threat of GHG emissions must take precedence over scenarios which are 100% hypothetical.
@Leo Hansen
Even if you are right about glaciers and drinking water, there are other problems associated with glacier melt, including flooding with possible dire results for local populations. This is close to happening in the Himalayas
http://www.telegraph.co.uk/earth/environment/8538974/Everest-glacier-turning-into-a-lake-due-to-global-warming.html
However, this is veering into an AGW/CC discussion and the Mod might rap us on the wrist for it soon – so maybe we should continue the conversation elsewhere on the blog.
MODERATOR
This is veering off track – it is only running in this thread because of Tom Blees’ inclusion of glacier melt in his article -please move to Open Thread 15 if you wish to continue.
Sod said:
“we need reactors that will work while the coolant is leaking out.
A single disconnected assertion has been presented as though it is meaningful.
Coolant is not an optional extra. It is part of the whole show. If it is leaking out at a rate that reduces the ability of the reactor to perform its function safely, then what is needed is a safe shutdown option and a containment system. To assert that a power station should be able to operate without coolant is analogous to asserting that cars must be able to run without coolants – oil and water. Of course they should be able to be able to be brought to a halt, but to continue running without coolant?
So, Sod: A question for you. What current design of NPP does not include those two features: containment and safe shutdown following loss of coolant? I’m no expert, but I’ll hazard an answer.
The answer is: None. None at all. Zero. Zip. Nada.
The question is a straw man.
The hypothesised circumstances do not exist.
Sod’s rant was entirely pointless.
As to the reasons for Germany’s planned phasing out of its NPP’s… let their actions be consistent with their words. I will believe Angela Merkel’s commitment against NPP’s only if and when she advocates symultaneously cutting the power lines which join Germany to its neighbouring nuclear powered states. Until then, we are not discussing anti-nuclear commitment, we are discussing populist green luddism via the ballot box and the effect that this can have on politicians who seek to be seen to be acting, whilst not really acting at all.
If the Germans actually do shut down those reactors, I am pretty sure that new, larger reactors will be built on the very same sites soon after, when the folly of their decision becomes undeniably self-evident.
Environmentalist:
“Yes I know just nameplate capacity.”
This quotation indicates the shallowness of the reasoning at work. The author agrees that the basis of comparison is false, yet he persists in his claim about the relative weights of nuclear and renewables post-Fukushima.
Once the BS factors have been adjusted out of the nameplate ratings of renewables and the productive capacity has been substituted, the claim has been falsified.
“Falsified”, dear Environment, is science talk for wrong, discredited, untrue.
Your statement is, by your own admission, wrong, discredited, untrue.
Well done.
Hank,
Re. Cassandras, I plead innocent on that one. Barry actually posted an older version of the article. I realized the error and had changed it to doomsayers (see the pdf) before it was submitted for publication.
C. Monneron,
That measurement of ground acceleration sounds exceedingly, even unrealistically high. I’m going to run that by a friend of mine who’s a geophysicist and I’ll let you know what he says about it.
Tali, the core meltdown expectation from risk assessment studies is meant to reflect the equipment failing, not purposeful human intervention/sabotage. But that does raise the point of just how easy subterranean PRISM reactors (at IFR sites) would be to protect from terrorist actions. If ingress to the reactor was only possible through remotely-operated blast doors, it would be a very unlikely thing indeed.
Greg M: I’ve seen the $1,000/kW cost for future Chinese reactors bandied about several times, but just off the cuff I found this—tinyurl.com/4xlz3dr—where it says they plan to be able to build AP-1000s for $1,100/kW after building 50 of them. I believe PRISMs or another such non-pressurized modular fast reactors could easily match or exceed that, especially if they employ supercritical CO2 Brayton cycle systems, which at this point look like they’ll be not only way smaller but would boost power conversion rates by 50%, meaning you could build a single power block of 2 PRISMs that would produce a gigawatt of electricity.
Max, Barry is planning to do a post in the near future on why sodium is the preferred coolant. When I was researching my book I did my best to find out a better way, but sodium has compelling advantages and it simply isn’t prohibitively difficult to engineer the system for extremely safe operation (you don’t put water in the same structure as the reactor vessel and you use an argon atmosphere). As for being able to produce hydrogen with higher temperatures (high-temp reactors having their own set of problems and not being deployable yet, unlike sodium), who needs them? If we build enough IFRs to meet our electrical needs, that means we’d have enough generating capacity to meet peak demand. But that’s only about 1-2 hours/day. All the rest of the time you could use the extra juice (they don’t mind running at full bore 24/7, and the fuel is essentially free) for electrolysis, desalination or whatever else. I suspect the H2 from the electrolysis would be used to make ammonia for vehicle fuels, all completely doable with the abundant surplus power.
Environmentalist writes: “IFRs have been tried and abandoned, superphenix being the most notable failure, the constant sodium fires made it intermittent, unreliable, expensive, and dangerous.”
You’re not actually talking about IFRs but SFR (Sodium Fast Reactors). The Superphenix was a FOAK that admittedly had its problems at first, but the majority of its problems were political, and it ran just fine the year prior to it finally being shut down for political reasons. The French ran the smaller Phenix for over 30 years very well, as have the Russians with their BN600. IFRs per se have never been built on a commercial basis, but the IFR at Argonne ran for over 30 years very well.
And then: “Yes I know just nameplate capacity, but with Nuclear slowing down after Fuskushima, and renewables rising, its only a matter of time before 50% of all new powerplants per kWh of yearly energy is renewables.”
Call me when you get there. I’ll buy you a beer.
Thanks Tom.
Just 2 questions for Tom or anybody else who might know.
1) How much energy are you talking about in that golf ball of depleted uranium?
2) How much coal would need to be mined for the same amount of energy?
@Joffan:
You are right, I was confused.
I confused two reactors: EBR-1 was sodium-cooled and had a meltdown ; SL-1, at the same lab (Idaho), was water cooled.
Apologies for confusion.
@Geoff Russell:
1) Assuming a 43 mm golf ball, and 50% conversion efficiency — about 9 million kWh. (200 MeV is about the energy of a fission; 19.1 g/cm^3 is the density of uranium. U-238 weighs ~238 grams/mole.)
2) Around 3,000 tons, depending on the type of coal and power plant efficiency.
““Falsified”, dear Environment, is science talk for wrong, discredited, untrue.
Your statement is, by your own admission, wrong, discredited, untrue.”
Well if that were the case then the 375 GW of installed capacity in Nuclear is also wrong, discredited, untrue as its energy output per year is ~90% of 375 GW * 24 h * 365. Imagine the faces of hypothetical people that planned around expecting 3285 TWh…
I still do not understand the hostility of normalizing power output with the expected capacity factor, sure there are many that just look at the nameplate but nobody here does.
“You’re not actually talking about IFRs but SFR (Sodium Fast Reactors). The Superphenix was a FOAK that admittedly had its problems at first, but the majority of its problems were political, and it ran just fine the year prior to it finally being shut down for political reasons. The French ran the smaller Phenix for over 30 years very well, as have the Russians with their BN600. IFRs per se have never been built on a commercial basis, but the IFR at Argonne ran for over 30 years very well.”
They all have the same issues, Sodium is extremely reactive/explosive when coming into contact with the water that is supposed to turn to steam. Could the engineering fix this problem? maybe but I will believe its economically possible, and safe, when I see it.
“Call me when you get there. I’ll buy you a beer.”
Very well
A while ago, Barry posted this link:
http://features.blogs.fortune.cnn.com/2011/03/24/whats-next-for-nuclear-power-nassim-nicholas-taleb/.
The main argument is that statistical models don’t work for rare events and therefore statements like “one meltdown in X years” are not
credible.
At least from a philosophical point of view that seems correct: we can’t make predictions without enough observations.
So why are people still talking about “one core meltdown once every 2900 years”?
@Geoff Russell — “How much coal would need to be mined for the same amount of energy?”
A short, sharp reply to the anti’s is: “well, would you prefer one tonne of fission products deep underground, or a million tonnes of CO2 in the greenhouse?”
This tactic shifts the focus of debate to the much greater threat presented by a damaged greenhouse. Ideally, it’s more than one million, but there’s no time to split hairs in a shouting match.
uvdiv: Thanks … coming at it from the other direction …
http://en.wikipedia.org/wiki/List_of_countries_by_electric_energy_consumption
US electricity power = 1.4 kw per person.
1.4*365*24*70 years = 858480 kwh, multiply
by 3 or 4 to get total energy … so okay 9e6 kwh has plenty of slack!
Roger: Good argument!
Following
Tom Blees said:
‘Only 20,000 years ago the Laurentide glacier covered most of Canada and over a quarter of the USA; it extended a mile deep to where New York City is today.
Farmers in Canada and America’s heartland are shedding no tears for the departed glacier.’
Our descendants aren’t likely to be farming where the Greenland and Antarctic ice sheets were: those areas will still get half year days and nights.They might cry a bit about the flooding of most of today’s cities and plains though.
@ Hank Roberts: yes the Japanese plant added a hardened vent and some other modifications – BUT they did not make the most important change that were made for US BWRs: the vent still rejects to the upper building, where hydrogen can accumulate. US plants changed this to external chimneys so that the upper building portion wouldn’t be damaged in the event of hydrogen detonations.
I don’t like the industry response of “we want to be able to use a valve so we can turn it off when we want”. This caused Three Mile Island – operators ignored alarms and closed critical valves for cooling. When operators are under stress they make mistakes. We assume a 30% failure rate for operators under severe stress of accidents, for industrial installations, in our PRA modelling. This is not something you want to rely on for ultimate barriers.
For ultimate safety, use rupture discs. When they go it means you have a severe accident already since you’ve exceeded the containment design pressure at which the rupture discs will burst.
It appears my question related to filter systems remains unanswered; the Fukushima vents clearly didn’t have carbon and high U class HEPA filters in the emergency vent lines. That’s a design flaw.
Re energy density, here’s my take on it:
http://energyfromthorium.com/forum/viewtopic.php?f=39&t=2757
Thorium in an isobreeding MSR or uranium in IFR is , by volume, twenty to thirty MILLION times more energy dense than fossil fuels. Compared to burning wood its over two hundred MILLION times more energy dense.
Tali, on 29 May 2011 at 4:15 AM: IF the terrorists managed to put a hole in a containment building, which is unlikely, that hole alone would not cause a radiation leak. The core is still inside a 5 inch thick stainless steel vessel inside the containment building.
Containment building:
The walls, ceiling and floor are a minimum of 1 meter [about 39
inches] thick and HEAVILY reinforced with steel. There is so
much steel reinforcing rod that when you look at one under
construction, you wonder where there will be any room for
concrete. The concrete itself is superconcrete, not the kind your
driveway is made of. There is no explosion that could ever
happen inside the core or the containment building that would
have any chance at all of making a hole in the containment
building. The containment building is many times stronger than
required to contain any explosion that could happen there.
Furherbunkers: When you are digging a hole to build a tall
building in Berlin, Germany and you encounter Hitler’s bunker,
you don’t try to destroy or remove the furherbunker.
Furherbunkers cannot be removed or destroyed. You just set the new building on the furherbunker. The same is true of American reactor containment buildings.
Likewise, there is nothing a terrorist could do to a nuclear power
plant that could cause a radiation leak. Just putting a hole in the
containment building would be pointless anyway. Radiation
would not leak out, but the reactor would be shut down while the hole was fixed.
Tali, on 28 May 2011 at 11:05 PM: The answer is: How in the world are they going to get inside the containment building? Your question is absurd. People don’t go there. If they do, the reactor shuts down automatically, triggering alarms elsewhere, many miles away. Even if they did blow a hole in the reactor vessel, they would accomplish nothing while killing themselves. The containment building would contain any radiation that got out of the reactor vessel, IF any radiation got out of the reactor vessel, which would still be unlikely. Go visit a reactor.
Recycling spent nuclear fuel: We used to do so in the old days. I almost took a job at Numec in 1968. Some of the spent fuel found its way to Israel, so the spent fuel became a hot potato.:
We don’t recycle nuclear fuel because it is valuable and people steal it. The place it went that it wasn’t supposed to go to is Israel. This happened in a small town near Pittsburgh, PA circa 1970. A company called Numec was in the business of reprocessing nuclear fuel. I almost took a job there, designing a nuclear battery for a heart pacemaker. [A nuclear battery would have the advantage of lasting many times as long as any other battery, eliminating many surgeries to replace batteries.] Numec did NOT have a reactor. Numec “lost” some nuclear fuel. It wound up in Israel. The Israelis have fueled both their nuclear power plants and their nuclear weapons by stealing nuclear “waste.” It could work for any other country, such as Iran or the United States.
It is only when you don’t have access to nuclear “waste” that you have to do the difficult process of enriching uranium. Numec is no longer in business. Terrorists can’t compete with Mossad and Israeli dual citizens who are CEOs of companies like Numec. Israeli nuclear weapons are exact duplicates of American nuclear weapons. All persons who were “born of Jewish mothers” are citizens of Israel regardless of any other fact. Since the US can’t and shouldn’t discriminate, the reprocessing of nuclear fuel in the US stopped. That was the only politically possible solution at that time, given that private corporations did the reprocessing.
My solution would be to reprocess the fuel at a Government Owned Government Operated [GOGO] facility. At a GOGO plant, bureaucracy and the multiplicity of ethnicity and religion would disable the transportation of uranium to Israel or to any unauthorized place. Nothing heavier than a secret would get out.
The problem is political: The Republicans think GOGO plants are socialist/communist, which is nonsense. A COCO [Contractor Owned Contractor Operated] plant is the low bidder, who is inevitably a front for Israel or some other country. We could send our spent fuel to France to be recycled. France recycles fuel for several countries. If we give it to Russia, the Russians will recycle it and sell it back at a much higher price.
See:
http://www.pittsburghlive.com/x/pittsburghtrib/news/specialreports/buriedlegacy/s_87948.html
Be aware that It couldn’t be weapons grade uranium. The US uses only plutonium to make bombs. A uranium bomb is primitive, lacking in sophistication, overweight and inefficient. Uranium bombs are for countries that are new to the club.
France and recycling:
http://www.ecolo.org
Association of Environmentalists for Nuclear Energy presents the following book.
http://www.comby.org/livres/livresen.htm
gallopingcamel: Before the glacier over Canada melted, there was MORE farmland. The continental shelves were above sea level.
Sodium: I prefer the fourth generation Modular High Temperature Gas Cooled Reactor (MHTGCR) with helium as the coolant and carbon as the moderator. It can’t melt down, it has no sodium, and it is very efficient.
Calculate your annual radiation dose:
http://www.ans.org/pi/resources/dosechart/
Putting shaped charges on an operating reactor vessel is impossible, the biological shield prevents you from doing this and even if you manage to get between the biological shield and the reactor vessel, the high radiation field would overwhelm you quickly (that thick reinforced concrete biological shield is there for good reasons!). But reactors are designed for large break loss of coolant accidents, whether a large pipe break or a hole in the reactor vessel. You’d have to also sabotage the diesels and pumps and various valves and emergency fire line injection systems. I really don’t see any way to do this without getting caught, passing out by high radiation fields, and shot by guards.
(comment deleted – off topic- please re-post on OT 15 or Sceptics thread.)
The dangers of sodium coolant may be exaggerated, but I prefer fluoride coolants for technical and economics reasons. Much higher heat capacity, transparent, low reactivity with water and air, higher temperatures possible. Here’s a paper by Charles Forsberg which is quite convincing.
http://arss.ornl.gov/reactor_design/icapp_2005_fast_reactor_Paper.pdf
Helium cooled designs are low power density, very high pressure. Not a good combination for economics and scalability.
The issue of the Brayton cycle also is not resolved. There are no Brayton Cycle Turbines of the closed designed in deployment, that I know of. It’s the *single* biggest stumbling blog to most HTRs. The Chinese abandoned it as well, using a gas/steam heat exchanger to drive a Rankine Cycle turbine. We need serious R&D on this.
(deleted – off topic – please re-post on OT 15 or Sceptics thread.)
We can get started with standard off the shelf steam turbines as used by modern coal and CCGT plants. These use steam of 550 to 600 degrees Celcius and are 44-46 percent efficient, already a big improvement over modern LWR turbines which are 37 percent efficient. It means one fifth more electricity per unit reactor (=thermal) capacity. When the gas Brayton closed cycles get ready these can be used. I doubt they would give a big efficiency improvement over modern steam turbines at the same temperature though (future steam turbines are being developed for 700 degrees Celcius steam, 50% efficient).
[…] Tom Blees writes in a guest post at Barry Brooks’ “Brave New Climate” blog about modern nuclear reactor technology. He observes that the fact of the Fukushima accident happening to a over forty year old design does not necessarily disqualify the security of modern nuclear technology. […]
[…] those less familiar with the technology to which I am referring, this article by Tom Blees will bring you nicely up to […]
Here is a presentation on the status of fast reactor and pyroprocessing development in Sth Korea:
http://www-pub.iaea.org/mtcd/meetings/PDFplus/2009/cn176/cn176_Presentations/plenary_session_1/FRP-05.Choi.pdf
Clearly a very close relative of the IFR. There are some interesting slides including projected 50 fold decrease in LWR spent fuel inventory by the end of the century (page 8) and projected use of super critical CO2 Brayton cycle turbines.
It also shows how advanced PWRs and SFRs may live together in a future reactor fleet.
I’m beginning to wonder if on-line discussions will ever produce tangible results. Probably not unless there is a large following or money is to be made.
Tali: Cyril R is also correct. Your question didn’t get answered for a while because your question is just too absurd. Some questions are so stupid that they can’t be answered. Sorry to use the word “stupid” but there isn’t a better word. Look at Figure 1. So you want the terrorist to dive into a pool of molten metal and swim down to the reactor core? Is that right? Can you see that people will question your sanity rather than bother to answer? There is really nothing a terrorist can do to a reactor except get himself killed.
There was a person on public TV who said that there are 13 federal regulatory agencies for nuclear power and 27 congressional committees to regulate nuclear power. Did I remember that correctly?
Cyril: I suppose I can guess what a “rupture disk” is … like on the top of my pressure cooker? What is it
precisely in this context … link?
Rupture disc.
Imagine a T-piece in a pipe under pressure.
A rupture disc on the branch would look like a circular metal blank inserted so that it blocks the flow, clamped between flanges.
At the rated pressure, the disc ruptures and the contents of the pipe vent via the branch line.
In a former life, I redesigned hydrogen systems at several power stations, adding rupture discs to each storage vessel and slightly higher rated discs to pipe routes, etc. Each disc was vented to atmosphere high on the exterior wall of the building. When the discs ruptured (very uncommonly, only after incorrect operation of the plant), the hydrogen spontaneously caught fire as it emerged via the vents. This was due to static electricity.
Rupture discs are mandatory on all hydrogen cylinders in Australia. One of my mods was to arrange them so that if they blew, there was no subsequent fire in an occupied space or affecting adjacent plant, such as the semitrailer which was caught up in the fire on one occasion.
They are low tech, reliable, cheap and effective. It is hard to argue against them, really.
Further designs will incorporate many more improvements to deal with radioactives gases and venting issues more easily, even without electricity.
I just hope to which extent we will be able to upgrade current design to deal with that kind of problem.
Germany’s coalition government has announced a reversal of policy that will see all the country’s nuclear power plants phased out by 2022.
I think this is great news because adoption of a definite time frame for the phase out will help to bring out the detailed planning of the highly disruptive and expensive build necessary to bring renewables online, and will also demonstrate, one way or the other, whether they truly do have a low carbon footprint: and, all the while, there will be the other model (predominantly nuclear) operating next door, in France, whose results they can compare with.
The rest of the world can watch developments with interest and we may just have enough time to learn from these outcomes and adopt whatever technology proves to be effective. This allays my main concern which was that no serious start was being made on finding the sustainable energy solution. Now there is at least a timetable as to when we will have the answer as to whether renewables can do the job or whether it has to be nuclear.
“So, basically, you’re saying we should not worry about what might happen to an LFTR, ESBWR or PRISM, etc., reactor in the event of an act of sabotage or war because there are a bunch of other things that people will blow up first because there easier or will kill more? How can you suggest that these reactors won’t have “military or political” significance when we’re talking about using them to replace the current role played by oil, coal and gas?” – Tali
In the case of the LFTR, the sabotage you suggest will probably be harmless to civilian populations. First, the preferred housing for LFTRs will be underground. Secondly, in order to operate as breeders, radioactive gases, including Xenon-135 will have to be removed from the carrier salts. In addition it would be an inexpensive safety measure to remove volatile fission products from the carrier salts. any attempt to blow up the LFTR will simply leave other radioactive fission products trapped underground. Carrier salts released by a sabotage explosion will simply drain into an underground dump tank. Fission products in the carrier salts will be tra[[ed both by chemical bonds and by the force of gravity.
Mullos i agree with your assessment. the conservative -liberal government has done serious damage to the development of alternative energy, by reversing the nuclear consensus formed by the social democrat -green government in 2002.
Development of a new technology depends on reliable conditions.
it is not easy to understand the new German exit from nuclear technology.
though the facts are simple: the 7 oldest reactors, which were already switched of in the moratorium will not go back online. in addition, the accident prone reactor Kruemmel will also not go back online. the remaining reactors will be switched of until 2022.
http://www.bbc.co.uk/news/world-europe-13592208
this sounds like a dramatic move, but if you check the old dates on this document, you will see that it is not very different from the social democrat – green plan in 2002.
http://de.wikipedia.org/wiki/Liste_der_Kernreaktoren_in_Deutschland
some reactors will run a little longer, other a year or two shorter. but basically it is the same thing under a different name.
the big difference is, that now the conservative and liberal party are also pushing for the exit. (we have some more elections coming up, and the basically lost all this year!)
so the biggest news is, that a reversal of the exit is extremely unlikely this time.
@Tali
How can you suggest that these reactors won’t have “military or political” significance when we’re talking about using them to replace the current role played by oil, coal and gas?”
We have a decades worth or targeting data.
The World Trade Center was a symbol of economic might, the Pentagon was a symbol of military might. Most of the rest of terrorism was soft targets. school houses, hotels, open air markets etc. Easy to plan and execute.
Blowing up a nuclear plant requires people rational enough to complete complex tasks and irrational enough to go on a suicide mission and suspend ‘self preservation’ reflexes. Not an easy combination to find.
It would be fun to see what happens when the silly German greens realise they don’t have enough electricity left and are faced with increasing fossil fuel consumption and/or blackouts/brownouts. That may be the only way to push the Germans back into a reality based energy policy (as opposed to living on fantasy island today).
Cyril R that is a very pessimistic prediction
“The announcement came as a Federal Environment Agency (UBA) report revealed that a rapid phase-out of nuclear energy would have only a modest impact on Germany’s economy.
Daily Frankfurter Rundschau reported on Friday that an assessment by the agency found that if all nuclear power plants were shut down by 2017, electricity prices would increase by just 0.6 to 0.8 cents per kilowatt hour and there would be “no significant loss” in economic growth.
A shut-down would “have substantial benefits and outweigh the modest increases in electricity prices,” the report said.
The report also said the withdrawal could be achieved without the risk of electricity blackouts because “sufficient surplus reserve capacity” exists.
”
http://www.thelocal.de/national/20110527-35293.html
This is the reserve capacity the speak of.
http://rwecom.online-report.eu/factbook/en/marketdata/electricity/grid/germanyimportandexportofelectricity.html
@ Mullos on 30 May at 10.04 PM.
That is somewhat contorted reasoning you display.
We don’t need German stupidity to demonstrate the impossibility of relying on renewables for base load power. Simple reasoning based on first principles makes this obvious.I doubt whether the present German government,with its political reliance on the idiotic Green movement,will last too much longer anyway for various reasons. I do give the German people some credit for common sense.
Furthermore,we don’t have the time to wait on the results of the German adventure if it,by some mischance,is allowed to continue.
@sod
“Development of a new technology depends on reliable conditions.”
I would add that development and deployment require reliable conditions. That’s one of the reasons why the French were more successful than others for several decades. This 36 page study argues that the french deployment was successful above all else because of a “unique institutional setting allowing centralized decision-making, regulatory stability, dedicated efforts for standardized reactor designs, and a powerful nationalized utility, EDF, whose substantial in-house engineering resources enabled it to act as principal and agent of reactor construction simultaneously.” It also draws a number of other conclusions that are worth pondering.
http://www.slideshare.net/myatom/costs-of-the-french-pwr
The German’s will not suffer blackouts as a result of the closure of their nuclear plant. This whole farce was orchestrated to remove the last real competition to Russian gas. All of the nuclear generation lost will be replaced by generators burning NG, with a few windmills and solar to provide the illusion that they are following the green path.
The big winner here is Gazprom, not the Greens
Thanks John.
DV8 is 100% correct. They are well meaning dupes, unfortunately. See this and the commentary:
http://www.dailykos.com/story/2011/05/30/980516/-Germans-abandon-Big-4-Energy-Companies-in-drovesGovt-to-Close-Nuke-Plants?via=search
sod, on 31 May 2011 at 1:02 AM
The reversal of the exit is not “extremely unlikely” but impossible.
This is irreversible. Only if they built a new reactor, which is unlikely.
It appears that the Electric Power Research Institute (EPRI) has recently released a study of the so-called smart grid. The study cliams the ‘smart gridding’ will cost half as much as traditional grids to install over the next two decades in the USA,
Someone might care to find a copy for a critical analysis.
Here is an article based on the EPRI summary (I think):
http://eponline.com/articles/2011/04/07/benefits-top-costs-in-epri-smart-grid-analysis.aspx
At the end there is a link to the EPRI report.
@ Sod, on 31 May 2011 at 1:02 AM:
The first of Sod’s two links leads to an RWE slide show “Facts and Figures, 2008. The particular slide which SOD cherry-picked was #36. He claims, in a roundabout way, that there will be no problem if Germany cuts off 7 NPP’s early, then follows up with the remainder of its stock – 22% of its generating capacity overall.
Slide #3 demonstrates that 300,000 MW of new generation capacity will be needed in Europe by 2020 and even more, beyond then. The figure becomes 50% of total power by 2030. Where will this new 50% come from, or will Germany make do while supplying only half of its national demand for electricity?
@ Environmentalist, on 31 May 2011 at 4:53 AM:
Enviro stated “if all nuclear power plants were shut down by 2017, electricity prices would increase by just 0.6 to 0.8 cents per kilowatt hour and there would be “no significant loss” in economic growth.
His source for saying “sufficient surplus reserve capacity exists” is the very same RWE.
Sod, RWE and Enviro cannot have it both ways.
A shortfall of 300,000 MW installed capacity is most definitely not a description of a situation with surplus reserve capacity, or one with a growing economy with stable energy prices.
Come on, fellows… at least read and understand that which you are quoting before you bring it here.
The Germans will very much miss the nuclear 22% of their 2008 generating capacity and the remainder of Europe will not be able to make up the shortfall.
An over-optimistic do-nothing approach is not going to work; Germany is digging a very deep hole for its industries, its balance of payments and its households. The crunch will come in 2017, when the remainder of the German NPP’s is retired, if not earlier.
Note well: These comments have been prepared using data from the very same source that Environmentalist and sod quoted. Shot with their own bullets.
John Bennetts, on 31 May 2011 at 11:23 AM — I don’t think they’ll be able to afford bullets; arrows perhaps?
David Benson: The EPRI article you referred to is a free 6.5MB download.
I am experiencing difficulty connecting to the site, but that’s because I live in the bush, where NBN will probably never reach me.
The EPRI document downloads OK.
“A shortfall of 300,000 MW installed capacity is most definitely not a description of a situation with surplus reserve capacity, or one with a growing economy with stable energy prices.
Come on, fellows… at least read and understand that which you are quoting before you bring it here.”
They do take it into account, Germany and Europe for that matter will not be remaining static, they will keep adding renewable energy into the grid 300 GW seems feasible actually for all of Europe in 10 years.
I find the similarities between what is happening with Germany post-Fukushima, and Italy post-Chernobyl rather compelling.
Italians in 1987 decided to phase out nuclear, and did so by 1990. Later it was claimed that it was a terrible mistake and cost approx. 50bn Euros. The Italians had to resort to importing French Nuclear power. Bloomberg yesterday reported that the French became a net exporter of electricity to Germany in April, which is out of the norm.
Factor in the opposition to building large high voltage lines accross the Southern German forests to bring down the renewable power fom the North, and the French option seems like the only option politically. Not good for Energy Security when you have to rely on other countries for your electricity generation, especially Russian Gas (if that is the case).
The parallels that are being exhibited with the Italian retreat in the late 80s/early 90s and the current German retreat from Nuclear power are rather compelling. Seeing as France in both cases is exporting to overcome the shortfall. I wonder if after 10 years of the total phase out the German Chancellor will proclaim “It was a terrible mistake”.
Apologies if I broke any posting rules, first post and all.
In a way Environmentalist is correct: they are not committed to a truly massive build out of solar and wind. This will immediately effect their budget as the subsidies will become overwhelming.
The fact is that Gasprom and other natural gas companies are expecting to make huge gains out of this. There is already a huge gas turbine construction plan underway.
They will “make it” to this 40% level, at a huge cost, and by basically exporting some of their nuclear to France and the Czech republic. If I were France I’d double my nuclear build because Switzerland is going to be a worse situation as 40% of their grid is nuclear.
Politically, the UK, Sweden (so far), Finland, France and the Czech republic ought to form a Euro-nuclear consortium. They could help each with finance and engineering so they can absorb the generation countries like Spain, Italy, Germany and others are going to need to support these countries “going ‘green’”. This way they can be in the drivers seat.
It would reduce these countries to generation neo-colonies of the nuclear producers.
@Environmentalist, the only thing that is going to be added of any real consequence to the European grid in the next ten years is more Russian gas. Ever last bit of wind and solar added will only be there to provide a green veil to hid it.
Why you and other supporters of wind and solar steadfastly refuse to see that this is what is happening, is beyond me. You are being used by FF interests as auxiliaries in their fight. The funny thing is that even if wind and solar could assume 100% of the load, those same interests wouldn’t let it. They are not supporting renewables because they are good citizens – they are doing it because it’s good for their bottom line.
For Christ’s sake, wake up and smell the coffee.
David, you beat me to the punch.
IT IS NECESSARY TO ANSWER STUPID QUESTIONS because if we don’t, the wrong answer may be assumed by someone who knows nothing about it but who is very good at pushing people’s emotional buttons. Likewise, it is necessary to tell an innumerate humanitologist that she is wrong and how and that she should get a degree in physics or nuclear engineering before commenting on that subject. “You are being too emotional” is not an adequate answer. We need to really explain the truth.
Just complying with making nuclear power safer has never worked. We must explain that coal, wind, solar etc are far more deadly and that not having electricity is the most dangerous thing of all. Otherwise, you are on an infinite treadmill of making nuclear safer and gaining nothing. We need to get corporate money involved in advertising the fact that nuclear is the safest source of electricity. This includes teaching everybody about natural background radiation. Teaching everybody about natural background radiation does work.
After 100 responses on this thread, I’m outa here.
I think the best way to promote nuclear is to let the German experiment unfold but have France and Czech republic boycott sale of electricity to Germany. Give it 5 years of green utopia (deleted ioffensive remark)
(Rick’s offensive remark deleted)
Back on topic, the Germans are indeed looking down the barrel of a F-up. They might learn the hard way the importance of reliability.
Right again DV8.The kindest thing to be said about Germany on the energy score is that they are somewhat confused. The only way they’ll replace the 23.8% lost from nuclear phase out will be for an increase in fossil fuel use [gas]. That will be marginally better than coal but still not emissions-free. I thought that was the whole purpose [emissions-free] of our future power generation. Oh yeah, I forgot the renewables. There’s no hope of an adequate 24/7 supply with them, not ever, ever. Got that.
@Podargus, on 31 May 2011 at 7:00 AM
Unfortunately, “we” are not the ones who need to be convinced about the impossibility of relying on renewables for base load power. It is the general public and their governments. A constant complaint from the pro nuclear lobby is that excess regulation against nuclear and subsidies in favour of renewables are tilting the playing field. Obviously the renewables supporters have succeeded in getting the ear of governments to have achieved this.
The reasoning against using renewables might be “simple” but, by itself, it is obviously not enough to convince even a country like Germany with a long and impressive record of technological innovation and expertise. I think that what is at work is a mixture of entrenched core belief in the evils of nuclear and an optimism generated by the growth of “people power” successes which give rise to the attitude that somehow someone will come up with a new development to solve this problem. The renewables supporters are very fond of the argument that their costs are reducing and assert that they will solve the zcalability and cost problems if only more and more can be invested in R&D.
But, as Podargus has said, we don’t have the time to wait for a new silver bullet to emerge. Otherwise, we will be stuck with the renewables outcome which David Mackay rails against in “Sustainable Energy Without the Hot Air” —If everyone does a little, we’ll achieve only a little”
Somehow, people have to be convinced to discard the superficially attractive renewables and go back to that which they have previously set their minds and hearts against. Governments will quickly respond if public opinion moves in that direction.
Achieving such a reversal will require a concrete demonstration of the superiority of one system other the other, theories or modelling won’t be enough.
I expect that it won’t take till 2022 for the result to be clear cut. Germany will have to develop a clear detailed plan now to achieve the phase -out so the consequences will start to hit home as its citizens are told what they will be giving up to achieve 10 % efficiency savings, where the new pylons are to go, how much their power bills are to rise relative to France’s etc. Already, some commentators on this site are saying 5 or 6 years should be enough.
That may still be longer than what we would like, but I think it is shorter than what could have been achieved without this development.
Mullos well said. The only other way public opinion will change and thus government policy, is a global climate event that is dramatic. However this may be 20 years or more away and will be too late to stop warming of 2 to 4 degrees C by the end of the century.
I’m looking forward to the post on sodium coolant.
I do believe that the debate of lead vs sodium is by no means closed. According to a report* which studies both coolants, lead-cooled fast reactors are safer, but sodium-cooled fast reactors are minimally more efficient at burning actinides and a more mature technology.
From an operational security and public acceptance viewpoint I believe that if fast reactors are commercialized in a Western country they will be lead-cooled. Even if they don’t hurt anyone, sodium fires do disrupt the operation of the power plant.
*http://www.sciencedirect.com/science/article/pii/S0029549306003347
Germany’s Nuclear Ban: The Global Effect
“Germany’s plan to shut all of its nuclear power plants within the next 11 years will send waves — not ripples — through the energy industry and the offices of policymakers throughout the world. In short, Germany’s nuclear ban is a global game-changer.”
“In the short term, Germany, most likely will import nuclear power from France and the Czech Republic. This will place pressure on the existing nuclear power supply and drive up costs as a result. .”
“Germany’s nuclear ban will add about 25 million metric tons of CO2 emissions a year. “
“Today, renewable energy provides about 13 percent of Germany’s power. By 2020, it wants renewable sources to provide 35 percent of its electricity. To be clear, it will take a massive effort to reach that goal.”
“If it fails, governments that have been slow to embrace renewable energy will use Germany’s problems as a reason to backpedal from the source altogether.”
On the issue of sodium coolant, my understanding of it is that in most breeder reactors there are two coolant loops.
The primary loop that cools the reactor directly, then there is the secondary loop that takes the heat out of the primary containment to the steam generators and that’s where the problem is.
If the steam generators develop a leak and catch fire you have to shut everything down for repairs and even though there’s no danger to the reactor itself this still ends up probably being a significant economic cost.
Leaving aside the notion that it very well might be possible to develop a sturdy and reliable sodium-to-water heat exchanger with a little engineering and elbow grease the thought that always occurs to me is:
If we’re that worried about it, why don’t we try to find something that doesn’t react with sodium or water and use that as the coolant in the secondary loop? That seems a bit more reasonable than throwing out decades of work and research on an otherwise promising reactor.
Nick this has been my primary concern about sodium reactors all along. Sodium is an otherwise fine material except for that darn water sodium interface. (deleted personal opinion presented as fact and unsubstantiated by refs.)
Gene:
Well, yes.
Current sodium-to-water heat exchangers do tend to leak now and then which causes problems.
I was agreeing with that.
For various reasons, some of which could be called pseudo-philosophical and are outside of the scope of this thread, I want to keep breeding ratios as high as possible. As far as I know this means metal fuel and sodium coolant in the reactor itself, so that’s the baseline I’m working from.
Which means either building super durable heat exchangers or eliminating the water-sodium interface.
The former may very well be possible, perhaps using diffusion bonded heat exchangers rather than tube-in-shell designs to eliminate the welds that are what tend to leak? There’s one company that makes such heat exchangers and indeed lists metal cooled reactors as a compatible application: http://www.heatric.com/index.html
Or the other option is make sure sodium and water never meet. I’m told lead-bismuth eutectic doesn’t react with water and has a reasonable melting point, if it doesn’t react with sodium either then perhaps that would be worth a try? Also, for all of the talk I’ve seen about using gas turbines for future reactors might it be possible to have a sodium-to-gas heat exchanger and just remove water from the mix altogether?
…
I guess all I’m trying to say is that sodium + water = fire shouldn’t automatically consign sodium fast reactors to the scrap heap.
Use of another material for the intermediate loop such as nitrogen or CO2 might be feasible. I often wonder why we need the intermediate loop. Isn’t there a safe design that could use just sodium and some other material other than steam for the turbine with just a single loop through the turbine? CO2 turbines are of interest because of their high power with respect to their size. Just because we have used steam for so many years does not mean we have to use steam forever. I’m obviously way over my head on this topic. I wish I also had a degree in nuclear engineering….
You all do understand that at the sort of temperatures that are involved here, lead-bismuth erupting into water in a steam generator would be just as explosive as sodium. In other words, at 600-700C the amount of energy added by the chemical reaction would make little difference to the size of the explosion.
As well unlike sodium in the liquid state, lead-bismuth eutectic is highly corrosive to most metals making a rupture more, not less likely.
You might be interested to know that several non-nuclear power stations have been built that use mercury as a working fluid.
http://www.aqpl43.dsl.pipex.com/MUSEUM/POWER/mercury/mercury.htm
including the Clementine nuclear reactor
@Gene Preston
It is essentially because the primary loop gets activated, more than vapor in a BWR, so you don’t want the primary coolant to go out of the containment.
Lots of information from the horse’s mouth (I.e. Argonne)
http://www.ne.doe.gov/pdfFiles/SodiumCoolant_NRCpresentation.pdf
@DV82XL
from the referenced linked above :
heat generated from the reaction of Na with water :
140000 Joule
specific heat of one mole of Na @300C : 23*1.2= 27.6 J/K
140000/27.6 = 5072 K temperature difference !
From an instantaneous standpoint , chemical energy is not negligible.
It is not necessary to use sodium to water steam generators. There can be an intermediate loop fluid that transfers heat from the sodium to the water in the steam generator. A fluid that can’t be used in the primary loop because its not sufficiently stable against radiation but is compatible with both sodium and water. Certain fluorocarbons are a good example. A noble gas is also an option, helium for example. The latter being completely noble as an advantage, and high pressure, low volumetric heat capacity as a disadvantage (but this is not such a big deal for a steam generator that is non-nuclear and already high pressure on the steam side).
Of course, we’d be having four loops, which costs a bit and loses temperature in each loop.
First loop: sodium (activated, very radioactive)
Second loop: sodium (barely radioactive at all)
Third loop: helium (possibly final loop if helium gas Brayton is available) or fluorocarbon
Fourth loop: steam (if helium gas Brayton is unavailable).
I’m from germany and antinuclear forces are so strong here, right now you can’t win an election while supporting or even just keeping nuclear.
So, no matter if it’s a good idea or not, our government has to ban nuclear. It’s even a race who can demand the earliest ban. Many consider keeping it run till 2022 as a pro-nuclear position that plays on time and forgetting fukushima.
Also many people here think there is a big bad “atomlobby” financing all the arguments pro nuclear, so you don’t have to listen to them.
Here are some examples what the german climate&energy discussions look like (it’s in german):
http://www.heise.de/tp/inhalt/energie/default.html
It’s a shame.
@charles monneron -Consider the physics of a rupture of the sort we are contemplating. As the molten metal at such a high temperature hits the water, the water flashes to steam and the area in contact with the sodium is highly reduced, so most of the initial release of energy will be from this process, not the chemical reaction.
Since this will now be a liquid-gas interface, both the heat vaporizing the water and the evolving hydrogen from the chemical reaction will form a shield that will slow down the chemical reaction as the species won’t interact very well.
This is the same effects that has a lump of Na skidder around on the surface of a bowl of water rather than react all at once.
I am not saying a rupture would be without hazards, but it would not be much different if any molten metal hits hot water under these conditions.
The free enthalpy calculations don’t tell the whole story.
Cyril you have me thinking about another possible advantage of the intermediate loop. If the intermediate loop were either a gas or compressed liquid, might it not be possible to raise the temperature on the steam generator side, thus increasing the turbine efficiency? For example you could have a lower temperature sodium IFR reactor and then the intermediate loop would be inert, transfer the energy, and at the same time raist the temperature on the steam cycle side to super critical? I need Rod Adams to comment on this if you are monitoring Rod….
A gas compressor can increase gas temperature (and hence steam temperature on the other side of the steam generator) but it requires work to operate. You don’t get the work back because the work to be done is in the next loop (steam). You only get the heat. So unless I’m missing something this does not improve on the final work done. But if you have a turboexpander on the cold end it could be the most efficient means of moving the intermediate gas around. This isn’t an energy positive cycle though, and a fluid loop (using pumps) would always consume less work…
@DV82XL
I agree with you that the dynamic of the process is complex. Nevertheless, if the “steam envelope” tends to isolate the sodium from the water, it also lowers the heat transfer. At some point the sodium may boil and the sodium vapor interact with the whole volume of the steam or the steam/water interface. Ruling out an explosive dynamic is not obvious for me. It may also be dependent of the quantity and shape of the sodium sample.
My personal feeling is Merkel should have said ‘I support nuclear’ and been prepared to be defeated at the polls. She surely has the educational background to know, which I presume is why she initially supported nuclear.
The world is going to find out soon enough that nuclear is the only option. The only way it won’t be the only option is if a miracle occurs and, say, the polywell works, or some UFO lands on the WHL and gives us the secret to zero point energy.
My increasing feeling is that we are screwed. If even someone like Merkel, who really doesn’t need to win another election to afford to buy bread, can lie to us all about what it really takes to have a sustainable future, then I’d say we are in real trouble. The rot did not stop with Schroeder.
How I wish I was French.
@charles monneron, – The fact is that there have been sodium leaks, both intentional and accidental into steam generators that show this is not as big an issue as it might seem to be on paper.
The Japanese Fast Breeder Reactor MONJU had an event in December 8, 1995 as detailed here:
http://www.sozogaku.com/fkd/en/cfen/CB1011005.html
which was reasonably uneventful.
Nevertheless the physics of a breach in a sodium cooling loop is not simple, but it has been studied enough to understand the risks, and those risks can be controlled.
http://www.wpi.edu/Pubs/E-project/Available/E-project-022708-235336/unrestricted/MQP_Studies_of_Metal_Fires.pdf