Download the printable 33-page PDF (includes two appendices, on scenario assumptions and transmission cost estimates) HERE.
For an Excel workbook that includes all calculations (and can be used for sensitivity analysis), click HERE.
By Peter Lang. Peter is a retired geologist and engineer with 40 years experience on a wide range of energy projects throughout the world, including managing energy R&D and providing policy advice for government and opposition. His experience includes: hydro, geothermal, nuclear, coal, oil, and gas plants and a wide range of energy end use management projects.
Summary
Here I review the paper “Simulations of Scenarios with 100% Renewable Electricity in the Australian National Electricity Market” by Elliston et al. (2011a) (henceforth EDM-2011). That paper does not analyse costs, so I have also made a crude estimate of the cost of the scenario simulated and three variants of it.
For the EDM-2011 baseline simulation, and using costs derived for the Federal Department of Resources, Energy and Tourism (DRET, 2011b), the costs are estimated to be: $568 billion capital cost, $336/MWh cost of electricity and $290/tonne CO2 abatement cost.
That is, the wholesale cost of electricity for the simulated system would be seven times more than now, with an abatement cost that is 13 times the starting price of the Australian carbon tax and 30 times the European carbon price. (This cost of electricity does not include costs for the existing electricity network).
Although it ignores costings, the EDM-2011 study is a useful contribution. It demonstrates that, even with highly optimistic assumptions, renewable energy cannot realistically provide 100% ofAustralia’s electricity generation. Their scenario does not have sufficient capacity to meet peak winter demand, has no capacity reserve and is dependent on a technology – ‘gas turbines running on biofuels’ – that exist only at small scale and at high cost.
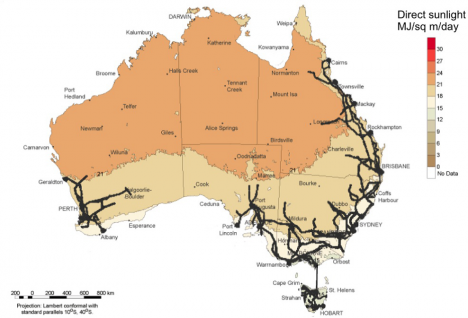
Map of Australia’s transmission lines. There are no transmissions lines to any of the proposed CSP sites, and the best solar areas are far removed from the existing transmissions infrastructure.Source: Grattan Institute, Figure 10.1 (attributed to DRET (2010), Grattan Institute)
Introduction
I have reviewed and critiqued the paper “Simulations of Scenarios with 100% Renewable Electricity in the Australian National Electricity Market” by Elliston et al. (2011a) (henceforth EDM-2011).
This paper comments on the key assumptions in the EDM-2011 study. It then goes beyond that work to estimate the cost for the baseline scenario and three variants of it and compares these four scenarios on the basis of CO2 emissions intensity, capital cost, cost of electricity and CO2 abatement cost.
Comments on the EDM-2011 study
The objective of the desktop study by EDM-2011 was to investigate whether renewable energy generation alone could meet the year 2010 electricity demand of the National Electricity Market (NEM). Costs were not considered. The study used computer simulation to match estimated energy generation by various renewable sources to the known hourly average demand in 2010. This simulation, referred to here as the “baseline simulation” proposed a system comprising:
- 15.6 GW (nameplate generation capacity) of parabolic trough concentrating solar thermal (CST) plants with 15 hours thermal storage, located at six remote sites far from the major demand centres;
- 23.2 GW of wind farms at the existingNEMwind farm locations – scaled up in capacity from 1.5 GW existing in 2010;
- 14.6 GW of roof-top solar photovoltaic (PV) inBrisbane,Sydney,Canberra,MelbourneandAdelaide;
- 7.1 GW of existing hydro and pumped hydro;
- 24 GW of gas turbines running on biofuels;
- A transmission system where “power can flow unconstrained from any generation site to any demand site” – this theoretical construct is termed a “copperplate” transmission system.
The accompanying slide presentation by Elliston et al. (2011b), particularly slides 5 to 12, provides a succinct summary of the objective, scope for their simulation study, the exclusions from the scope, the assumptions and the results.
The results of the baseline simulation show that there are six hours during the year 2010 when demand is not met, with a maximum power supply shortfall of 1.33 GW. It should be noted that the supply shortfall would be significantly greater with higher time resolutions, e.g. 5 minute data rather than the 1 hour increments used, but this limitation is not addressed by EDM-2011.
The EDM-2011 approach is more realistic than Beyond Zero Emissions (2010) “Zero Carbon Australia – Stationary Energy Plan” (critiqued by Nicholson and Lang (2010), Diesendorf (2010), Trainer (2010) and others), especially because EDM-2011’s approach, as they say, “is limited to the electricity sector in a recent year, providing a more straight forward basis for exploring this question of matching variable renewable energy sources to demand.” As the authors say, “this approach minimises the number of working assumptions”.
Despite the lack of costings, the EDM-2011 study is a useful contribution. It demonstrates that, even with highly optimistic assumptions, renewable energy cannot realistically provide 100% of our electricity generation. The baseline simulation does not have sufficient capacity to meet peak winter demand, has no capacity reserve, and is dependent on a technology – gas turbines running on biofuels – that currently exist only at small scale and at high cost.
The study is based on a number of assumptions that I argue are unacceptable:
- a system with insufficient capacity to meet the winter peak demand and no capacity reserve margin would violate Australian Energy Regulator (AER) requirements;
- the assumed capacity factors for the renewable energy generators are too high to be credible for the average plant life in a 100% renewable energy system;
- the assumptions about the way the existing hydro and pumped hydro facilities can be used are not practical;
- the assumptions about pumping and generating capacity of the pumped hydro plants are unjustified;
- the practicable capacity of gas generators running on biofuels (and the capability of the biofuel system to provide the fuel and store it until needed) has not been demonstrated and critical details are glossed over;
- the assumptions about a ‘copper-plate’ transmission system is unrealistic;
- the assumptions about reducing winter peak demand is highly optimistic and not borne out by recent experience.
These assumptions, and the cost of the system simulated are discussed in the following sections.
Comments on the technologies and assumptions
Gas turbines running on biofuels
Gas turbines running on biofuels are not a proven, commercially viable electricity generation technology at the scale required (IEA, 2007).
Although some countries, e.g. those quoted by EDM-2011, do have some electricity generated by biomass, there are a wide variety of technologies used, and very little of it is gas turbines running on biofuels. Much of it is in small plants, such as combined heat and power (CHP) fuelled by wood waste, chicken litter and other waste products. Most of it is in thermal plants, not gas turbines. IEA/OECD (2010), Table 3.7 lists four countries with some biogas capacity but this is mostly as reciprocating engine generators on waste dumps, sewage plants and the like. According to Energy in Australia 2011 (DRET, 2011a),Australia has 231 MW of biogas generating capacity.
The land area that would be required for the required biofuel production would be unacceptable (1.6 million hectares of prime agricultural land in good years (Electropaedia); far more in droughts; this represents 74% of Australia’s irrigated agricultural land and 4% of all arable land (ARNA, 2009)). The water requirements would also be unacceptable. As would the truck movements required to collect the biomass. A large commercial plant would need 100 to 200 truck movements per day and night collecting biomass from an area of 100 km radius (Simms et al., 2009)
The existing biomass electricity generation plants tend to be baseload or intermediate load plants. Some of the European biogas systems, which use a biomass feed, take around 30 days to make the biogas from the biomass feed. Such plants cannot be used for just the few days a year in winter when the CST, PV and Wind plants are unable to supply enough power to meet the demand. The biogas plants listed in IEA (2010) Projected cost of electricity generation, Table 3.7 have assumed capacity factors of 80%, 85% and 90%. These types of plants are not suited to the peaking plant role envisaged by EDM-2011.
Grattan Institute (2012) gives cost estimates for biofuel electricity generation inAustralia; however, the costs are based on a capacity factor of 70%. The report makes no mention of “gas turbines running on biofuels”. The technologies mentioned are steam plants and reciprocating engines. Following are three quotes from the report (Section 8):
For Bioenergy to provide 10% or more of Australia’s electricity needs it will have to use the large amounts of energy embodied within cereal crop residues
Even at 20 to 30 megawatts such plants require large amounts of biomass fuel to realise good capacity factors that are essential to offsetting the high upfront capital costs.
For a 30MW power plant at a 70% capacity factor the land area would be around 240,000 hectares and involve nearly 500 average sized wheat farms.
Note, these plants have to be run with capacity factors of around 70% to be economically viable. They are certainly not the sort of ‘peaker’ plants envisaged by EDM-2011.
For the gas turbines running on biofuels to work as envisaged by EDM-2011, I envisage biogas would have to be produced throughout the year and stored for use during the few days in winter at the times when the remainder of the renewable energy generators cannot provide sufficient power. The amount of biogas required per year is estimated to be 290 PJ (equivalent to 116% of natural gas consumed in electricity generation and 37% of total gas consumption in the eastern states in 2009-10). But most of this is required over just a few short periods in winter.
The cost of electricity from the biogas plants is crudely estimated to be $563/MWh based on the 13% capacity factor assumed in the simulations. Unlike natural-gas-fired gas turbines, which utilise low capital cost generators with readily available fuel, the biofuel proposal also requires capital intensive biofuel plants, year-round feedstock harvesting, and large-scale biogas storage and distribution infrastructure.
Given that the biogas option is so expensive, a cost estimate below was done for an alternative using natural gas instead of biogas. All other assumptions are unchanged.
However, even this alternative would be much more expensive than a system that uses gas throughout the year. In the baseline simulation, most of the gas generation would occur over a few short time spans each year. That requires either the gas supply lines be sized to deliver the gas volumes needed over the short periods, or the gas must be stored at site for use when needed. Either option will have a significant impact on the price of the delivered fuel and, therefore, on the cost of electricity. The baseline simulation has 24 GW of gas generation capacity supplying 28.1 TWh of electricity per year. However, EDM-2011’s Figure 3 shows that 26 GW is needed to provide a supply with no unserved energy and no unmet hours. This capacity in the EDM-2011 baseline simulation is about 4 times the capacity of the existingNEMgas generators.
We should expect the generators’ fuel costs would increase by more than a factor of four. One reason is that there is a small total consumption of gas over the year, but high usage rate for just a few short periods. The gas supply system would have to provide the infrastructure to deliver the peak capacity demanded, but it would be paid for by a small quantity of gas sold per year. So the gas price during the winter peak demand would have to be increased significantly. A second reason the gas price would increase is that there would be a much higher demand for gas in winter at the same time as the gas demand peaks for winter heating.
Hydro
EDM-2011 assumes the water could be saved through most of the year and used on the few short periods in winter when the renewable energy generators cannot meet the demand. This is not how our hydro schemes are designed to operate, nor capable of operating. Here are some reasons why they cannot be operated in this way:
- The generators would not be able to generate throughout the year to sell electricity at the time of peak demand. Therefore, their revenue would be much less over the year. So they would not be economically viable without a significant increase in the price they could charge for their electricity.
- The hydro generation is needed throughout the year to balance the power surges in the system. That is one of the most valuable functions of the hydro system and it will almost certainly be required to continue to serve that role.
- Hydro cannot be stored all year and released in a massive river flush over a few days in winter. To generate a great deal of energy over just a few days would mean large water releases which would compromise the management of storage and releases for irrigation and can cause flooding and unacceptable erosion to the river banks downstream.
- If the management of storage and irrigation releases is compromised the water would be released in winter and not available for irrigation in summer.
Hydro generation is constrained by the average water inflows and the water storage capacity to level out the fluctuation in water inflows over the long term. Snowy Hydro’s capacity factor is about 14%. Total generation by hydro in theNEMin 2009-10 was 12,522 GWh, and less in 2008-09 and 2007-08. This places an upper limit on the amount of hydro generation the simulation should generate.
It should be assumed the hydro generators will operate much as they do now.
Pumped hydro
The simulation assumes there will be no increase in the existing hydro and pumped hydro energy storage (PHES) capacity in the NEM. The existing pumped hydro plants have a maximum energy storage capacity of 20 GWh (Lang, 2010). There are also limits on the amount of energy that can be stored per hour and the time of day when pumping can occur.
The EDM-2011 simulation does not appear to limit the amount of energy that can be stored per day by the pumped hydro plants. I estimate the upper limit on the rate of storing recoverable energy with the pumped hydro plants is (MWh stored per hour):
Tumut 3 | 394 |
Wivenhoe | 328 |
KangarooValley& Bendeela | 157 |
Furthermore, there is a minimum duration for which the pumps must be able to operate continuously once started (e.g. 4 hours). So days when the pumps will not be able to run continuously for the minimum duration will not be able to store energy.
There is also a limitation on the hours of the day when pumping and generating can occur. They cannot occur at the same time. Since most of the excess power that would otherwise be spilled occurs during daylight hours when the CST plants are able to generate excess energy, it would seem that, in the simulation, pumping must be reserved for daylight hours when there is excess solar generating capacity.
It is not clear from the EDM-2011 paper how the model handles the distinction between the energy generated by hydro versus pumped-hydro in the two Australian facilities that are both hydro and pumped-hydro (i.e. Tumut 3 and Kangaroo Creek & Bendeela). EDM-2011’s Figure 2 shows pumped hydro generating at 2.2 GW for 40 hours on 9 and 10 January – a total of 88 GWh. This is not possible. There is only 20 GWh of storage and the pumps can store energy at about 4.5 GWh per day. The existing system would need to pump for about 7 hours with all pumps operating to be able to generate for 5 hours at 0.9 GW. So, the maximum daily generation, on consecutive days, would be about 4.5 GWh (excluding draw down from storage).
It would seem, with EDM-2011’s assumption of pumped-hydro being dispatched first, the 20 GWh of available storage would not be recharged each day since only about 4.5 GWh could be recharged each day. In the simulation, pumped hydro contributes little during the critical winter days shown in Slide 12 (Elliston et al, 2011b) and generates nothing on some days, e.g. July 1, 2, 5 and 6.
Only Wivenhoe is a ‘pure’ pumped hydro facility. The other two facilities are mostly hydro, with a small pumped hydro capacity. Therefore, it is more realistic for the EDM-2011 simulation to assume the hydro capacity is 6.6 GW and the pumped hydro can generate about 4.5 GWh per day at up to 0.9 GW on consecutive days (more for a short time if drawing down from 20 GWh of stored energy).
Concentrating Solar Thermal (Parabolic Trough)
EDM-2011 assumes a 60% capacity factor for CST. The details underpinning this are sparse, thus a number of questions arise. Is the assumed capacity factor a realistic average for the life of the plant? What is the basis for the assumed capacity factor for CST? Does it take into account:
- The system performance and reliability that is likely to be achieved over the full book life of the facilities?
- Spilled energy?
- Scheduled and unscheduled outages?
- Outages in the long transmission lines (which are mostly in remote areas far from the major service centres, so repairs will take longer than for the existing system)? Inevitably, these transmission lines will have lower reliability than theNEMaverage. Therefore, the capacity factor of the wind and CST plants would be reduced because of transmission line outages.
PV
What would be the average capacity factor for a fleet of 14.6 GW of roof-top, fixed plate PV over a 30 year life?
- How much would have to be spilled because the distribution system cannot handle the peak power output and power surges?
- How much would the assumed 16% capacity factor be reduced over the 30 year assumed life of each installation as a result of, for example:
- Performance deterioration of the solar panels
- Performance deterioration due to collecting dirt and lack of cleaning
- Some PV installations stop working or are disconnected, for whatever reason, and are never fixed or reconnected
- Buildings are sold, new owners are not interested in maintaining the system; some don’t keep it connected
- Buildings are knocked down and rebuilt without reinstalling the original PV system (the cost analysis assumes an average 30 year life for the original installations).
Is 14.6 GW of roof top solar PV realistic? That would be the equivalent of 1 kW for every man woman and child, or average of over 2 kW per dwelling. The PV is assumed to be on residential dwellings many of which could be on apartment blocks with limited roof space. Many of the houses may have tree shading and many will not have sufficient north facing roof space for a 2 kW system.
While the inclusion of 14.6 GW of rooftop solar may be theoretically possible, theNEMcould not accommodate such a concentrated non-dispatchable and variable energy supply without large-scale distributed storage and advanced ‘smart-grid’ management. All of which is expensive, but no attempt has been made to cost this
Wind
The assumed capacity factor of 30% for wind seems too high for a 100% renewable system. Although this is a valid figure for individual wind farms, much of the wind energy from a large-scale network of farms would have to be spilled. So the system wide average capacity factor for wind would be less than 30% in an all renewable energy system comprising primarily solar and wind generation.
Transmission
The EDM-2011 simulation assumes a ‘copper-plate’ transmission and distribution system (“power can flow unconstrained from any generation site to any demand site”). To achieve this assumption would require extensive additions to the existing transmission and distribution systems. The additions would need to have the capacity to carry the full peak power output from each generator plant.
The distribution systems would have to be upgraded to carry the peak power output of the PV systems in each area, or have smart grids to curtail the power output of the PV systems when they exceed the capacity of the distribution and transmission systems.
The additions to the transmission system would incur additional energy losses. Therefore, the 204.4 TWh of electricity generated in 2010 must be increased to account for the extra transmission and distribution losses. Appendix 2 contains more about the ‘copperplate’ transmission system assumptions, options and the basis for the cost estimates.
Winter peak demand reductions
EDM-2011 suggest methods to reduce the peak demand in winter so the renewable energy system can meet the demand. However, this approach is inconsistent with the stated objective which is to find a 100% renewable energy solution that can meet the 2010NEMdemand.
The relationship between energy efficiency and peak load is complex. As such, caution needs to be exercised in assuming that energy efficiency measures will invariably lead to commensurate reductions in peak demand. Indeed, electric vehicles and other unforeseeable new sources of demand may increase the peak.
Scenarios costed and compared
I have made a crude estimate of the capital cost, the Levelised Cost of Electricity (LCOE) and the CO2 Abatement Cost for the EDM-2011 baseline simulation. I have included an estimated cost for needed additions to the transmission and distribution systems to allow them to approach the ‘copper-plate’ assumption.
I have also analysed three additional scenarios with changes to some of the baseline assumptions. The changed assumptions include: sufficient generating capacity to meet all demand and maintain about 20% capacity reserve (which is less than a typical level for modern electricity networks, and much less than in theNEM); natural gas instead of biogas; reduced system-wide capacity factors for CST, PV and Wind, and less capacity for additions to the transmission system. The reduced capacity factors of CST, PV and Wind are compensated for by increasing the amount of generation by natural gas. Also included is additional generation to compensate for the increased energy loss in the additions to the transmission system.
The scenarios (detailed in Appendix 1) compared are:
- Baseline EDM-2011 simulation (i.e. gas turbines running on biofuels)
- Baseline with gas turbines running on natural gas
- Less renewable energy + more gas to improve reliability – Scenario 2 with most pumped hydro capacity reassigned to hydro, reduced pumped hydro capacity factor, reduced capacity factor of CST, Wind and PV, increased natural gas capacity and capacity factor.
- Reduced transmission capacity + more gas – Scenario 3 with half transmission capacity from wind farms, half transmission capacity of interstate interconnectors and reduced capacity factor of CST, PV, Wind and pumped hydro generation because of transmission constraints.
Capacity, capacity factor and generation assumptions
This section summarises the capacity, capacity factor, amount of generation contributed by each technology and each technology’s share of the total generation. These data are presented for the baseline (Scenario 1) and the three varied scenarios identified above as Scenarios 2, 3 and 4.
1. Baseline (i.e. gas turbines running on biofuels)
Table 1 lists the capacity, capacity factor, annual generation and share of total generation for each technology in the baseline scenario.
The capacity factors for hydro and pumped hydro energy storage (PHES) are not explicitly stated in the EDM-2011 paper. I have estimated the capacity factors for the baseline case by subtracting the energy generated by the other technologies from the total 2010NEMdemand (stated by EDM-2011 to be 204.4 TWh).
2. Baseline with gas turbines running on natural gas
Scenario 2 is the same as Scenario 1 but with the gas turbines running on natural gas instead of on biofuels. Table 2 would be the same as Table 1 except the ‘biogas’ column would be renamed ‘natural gas’.
3. Less renewable energy + more gas to improve reliability
The capacity, capacity factor, annual generation, and share for Scenario 3 are:
The total capacity is not the sum of the individual capacities because all but 0.5 GW of the PHES capacity is included in ‘Hydro’. The total generation is increased from 204.400 GWh to 214,600 GWh for an assumed 5% energy losses in the additions to the transmission system. The capacity of OCGT is increased from 24 to 33 GW to ensure 20% capacity reserve above peak winter demand. From Slide 12 (Elliston et al, 2011b), on July 1 peak demand is about 32.5 GW. At the time of peak demand there is little wind, no solar and no pumped hydro generation (because the pumped hydro was not recharged during the day). So, all the generation must be provided by hydro and gas. To maintain 20% reserve capacity (in case of unavailable generators) we need about 39.6 GW of gas and hydro capacity. We have 6.6 GW of hydro capacity, (excluding the 0.5 GW of ‘pure’ pumped hydro capacity because it may not have been recharged as was the case on July 1, 2, 5 and 6). So we need about 33 GW of gas capacity to give a 20% capacity reserve on1 July 2010.
4. Reduced transmission capacity + more gas
The capacity, capacity factor, generation and share for Option 4 are:
In this option the capacity of the transmission line from the wind farms is arbitrarily halved. The capacity factor and generation for wind is reduced because the transmissions line capacity is reduced. The capacity factor and generation for CST is reduced because the capacity of the intestate interconnector lines is halved, so less power can be transmitted from the solar plants, at times. The capacity factor and generation of PHES is reduced because the reduced capacity of the interstate interconnectors will reduce the amount of excess power that can be transmitted to and stored in the PHES facilities. The capacity factor and generation of OCGT is increased to compensate for the reduction in contribution from Wind and CST.
To clarify the differences between these assumptions for the four scenarios, the capacity of the technologies is compared in Figure 1, the capacity factor in Figure 2 and the annual generation in Figure 3.
Transmission and Distribution assumptions
For estimating the cost of the transmission system additions needed to achieve the ‘copper-plate’ assumption (Scenarios 1, 2 and 3), I assumed the transmission lines from each CST plant and wind farm will be sized to carry the rated power output of each facility. The transmission lines are assumed to run from the plant to the closest capital city or to the nearest entry point to the interstate interconnector lines.
The capital cities would have to be linked with interconnector transmission lines. For this crude cost estimating exercise I assumed their capacity must be sufficient to transmit the lesser of the peak demand at the receiver end or generation capacity minus demand at the sender end.
Figure 4 provides a graphic summary of the estimated capacities for the interstate transmission lines, as well as the renewable energy generating capacity (excluding biofuelled gas turbines) and the winter peak demand for each state.
For Scenario 4, the capacity of the transmission lines from the wind farms is half the rated capacity of the wind farms. The capacity of the interstate interconnectors is half the capacity assumed for the ‘Copper-plate’ scenario (shown in Figure 4). The capacity factor of the PV, CST and wind farms is reduced because of the transmission capacity constraint. Increased generation from gas compensates for the reduced generation from the CST and Wind generators.
The distribution system must allow the 14.6 GW of roof top solar PV, which is located in the residential areas, to supply their peak output without curtailment. It is assumed the transmission network would need to be ungraded to achieve this.
CO2 emissions intensity
Figure 5 compares the CO2 emissions intensity of the four scenarios with the 2010 NEMemissions intensity (DCCEE, 2010). The emissions intensities for the scenarios are for fossil fuel combustion only. Importantly, they are for gas turbines running on natural gas and operating at optimum efficiency. They do not take into account the higher emissions produced when the gas turbines are operating at less than optimum efficiency, for example during start up, shut down, spinning reserve, part load and when their power is cycling up and down to respond to changes in demand and changes in the output of the PV panels and wind farms. If these were included the emissions intensity for the three scenarios that use natural gas would be higher. They would also be higher if fugitive emissions were included. The emissions intensity figure for the NEMincludes fugitive emissions. None of the emissions intensities are life-cycle emissions so they do not include the emissions embodied in the plants. The emissions intensity used for the calculations is 0.622 t CO2/MWh ‘sent out’ (EPRI, 2010). See Appendix 1 for basis of estimates of CO2 emissions intensity.
Cost estimating methodology and assumptions
This section explains how the capital cost, Levelised Cost of Electricity (LCOE) and CO2 abatement cost for each scenario was estimated.
Except where otherwise stated, unit costs are derived from the Department of Resources Energy and Tourism (DRET, 2011b).
All costs are in 2009-10 Australian dollars.
Capital costs are ‘Total Plant Cost’ and do not include ‘Owner’s Costs’ and ‘Interest During Construction’ (IDC).
The inputs and intermediate calculation steps for each scenario are presented in Appendix 1.
Capital cost
Generation
The capital cost for each generator technology is the capacity times the unit cost ($/kW) for that technology. The capacity of each generator technology for each scenario is in Tables 1, 3 and 4. The unit cost for each technology, except gas turbines running on biofuels, CST and hydro, is the average of the high and low ‘Total Plant Cost’ in the DRET (2011c, 2011d) spreadsheets, converted to “sent out”. The central estimates are also presented in ACIL-Tasman (2010). The costs in the DRET spreadsheet are ‘$/kW installed’, so they must be converted to ‘$/kW sent out’:
$/kW ‘sent out’ = $/kW ‘gross’ / (100% – ‘Auxiliary Load %’)
DRET unit costs for CST are for 6 hours thermal storage. The EDM-2011 simulations assume 15 hours storage. The capital cost for CST is factored up by 1.53 to account for the increase of solar field and thermal storage size to increase energy storage from 6 hours to 15 hours. The factor of 1.53 was derived from the DRET (2011c) costs for CST without storage and CST with 6 hours storage, assuming a linear upscaling.
The DRET costs for PV are for 5 MW commercial installations. However, the simulations assume residential, roof-top, solar PV panels. These would normally be around 1 to 6 kW (say average 2 kW), not the 5 MW to which the DRET cost figures apply. The capital cost for PV should possibly be factored up by about 1.5 or 2. I have not done this in these analyses.
The DRET spreadsheets do not include ‘gas turbines running on biofuels’. There is very little commercial experience or cost information available for this technology. The capital cost and LCOE for gas turbines running on biofuels are based on $5,051/kW. This was derived from (IEA, 2007), IEA (2010), Grattan Institute (2012) and considerations of what would be needed to provide a secure supply of biofuels inAustralia. The cost estimate for gas generators running on biofuels has high uncertainty.
There is no capital cost for the hydro and pumped hydro plants because they already exist and there are no plans in the EDM-2011 baseline or the additional scenarios to build additional hydro plants.
Transmission additions and distribution enhancements
The capital cost estimate for the transmission system additions is the product of the transmission line length, the transmission line capacity and the unit cost ($/MW.km). The unit cost for additional transmission lines is estimated at $1,500/MW.km. This is derived from the AEMO (2011) cost estimates for the South Australian Interconnector feasibility study assuming a mix of AC andHVDC transmissions lines. The cost estimate assumptions and intermediate computation results are presented in Appendix 2. The largest uncertainty is in the transmission line capacity for the interstate connectors.
The capital cost for the distribution system enhancements to carry the PV generation is estimated at 20% of the asset value of theNEMdistribution system.
Cost of electricity
The Levelised Cost of Electricity (LCOE) for the generator technologies was calculated using the NREL LCOE calculator. The capital cost and capacity factor for each technology and each scenario are in Tables 1, 3 and 4. The other input values are as per DRET (2011c, 2011d) spreadsheets for all except the gas turbines running on biofuels, hydro and pumped hydro. Table 5 lists the other inputs.
The estimates of LCOE for generation using gas turbines running on biofuel assumes capital costs of $5051/kW (‘sent out’) and fuel price of $10/GJ to account for the costs involved with production, storage and transport. All other inputs for calculating LCOE are the same as for natural gas fuelled OCGT.
The assumed LCOE for hydro is $50/MWh and for PHES is $300/MWh[1].
The LCOE for the additions to the transmission network were calculated using the NREL calculator. The inputs are the capital cost (estimated as described above and shown in Figure 7) and the O&M costs. The O&M costs were estimated from the 2010 NEMO&M cost for transmission factored in proportion of the line length of the new additions compared with the total length of existing NEMtransmission lines (AER, 2011). Book life was assumed to be 40 years and discount rate as per Table 5.
The LCOE for the enhancements to the distribution system assumed the capital cost to be the equivalent to 20% of the 2010 value of the NEM’s distribution system assets. The O&M costs are assumed to be 20% of the NEM’s 2010 O&M costs (AER, 2011).
Costs not included in the cost estimates are:owner’s costs and interest during construction
- biofuel generating costs may be understated
- higher costs for natural gas to include the cost of building larger capacity gas pipes to supply 24 to 33 GW of peak gas generation (depending on the scenario), but with only 13% capacity factor to pay for the pipes (this means higher gas prices would have to be charged to pay for the high volume gas pipe system but with gas sales much less than the pipes could deliver).
- Increased O&M costs for CST with 15 h storage instead of the 6 h for which the DRET O&M costs apply.
- Costs for solar PV are probably too low (for kW sized, roof top, solar PV).
- Cost of electricity for the existing NEM transmission and distribution network. (Only the cost of the transmission additions and distribution enhancements are included. If the LCOE for the existingNEM network was included it would increase the cost of electricity for all options and make no change to the capital cost or CO2 abatement cost.)
CO2 abatement cost
The CO2 abatement cost is the cost to reduce emissions intensity from the CO2 emissions intensity in theNEMin 2010 to the emissions intensity that would exist with the new scenario implemented; it is expressed as ‘cost per tonne CO2 abated’ ($/t CO2).
CO2 abatement cost = (LCOE2 – LCOE1) / (EI1 – EI2)
Where:
LCOE1 = LCOE for theNEM in 2010
LCOE2 = LCOE for the scenario
EI1 = Emissions intensity for theNEM in 2010
EI2 = Emissions intensity for the scenario
The LCOE and CO2 emissions intensity for theNEMin 2010 are taken as:
LCOE1 = $45.40/MWh (AER, 2011; Chapter 1, Table 1.4)
EI1 = 1.0 tonne/MWh (DCCEE, 2010, Table 5, weighted average forNEM)
The LCOE and CO2 emissions intensity for each scenario are in Appendix 1 (and charted in Figure 5 and Figure 6).
The inputs and intermediate calculation results for the CO2 abatement cost estimates are in Appendix 1.
Uncertainties in cost estimates
The greatest uncertainties in the cost estimates are in:
- the fuel costs, capital costs and O&M costs for the gas turbines running on biofuels,
- the cost of the solar thermal plants with 15 hours of thermal storage and their lifetime average capacity factor, and
- the amount of additional transmission and distribution capacity needed.
Results
Capital cost, LCOE and CO2 abatement cost of the scenarios
Figure 6 compares the four scenarios on the basis of capital cost, cost of electricity and CO2 abatement cost.
Figure 7 compares the capital cost and cost of electricity for the ‘copper-plate’ additions to the transmission system (Scenarios 1, 2 and 3) and the scenario with reduced additions to the transmission system (Scenario 4).
Discussion
General
The EDM-2011 study reveals a great deal about the difficulty and cost of a largely renewable energy electricity system forAustralia’sNEM.
The study is more realistic than Beyond Zero Emissions’ “Zero Carbon Australia – Stationary Energy Plan” (critiqued by Nicholson and Lang, 2010; Diesendorf, 2010; Trainer, 2010; and others), especially because their approach, as they say, “is limited to the electricity sector in a recent year, providing a more straight forward basis for exploring this question of matching variable renewable energy sources to demand.” As the authors say, “this approach minimises the number of working assumptions”.
Despite the lack of cost estimates – a deficiency rectified in this paper – the EDM-2011 study is a useful contribution. It demonstrates clearly that, even with highly optimistic assumptions, renewable energy cannot realistically provide 100% of our electricity generation with currently available technology. The baseline scenario does not have sufficient capacity to meet peak winter demand, has no capacity reserve and is dependent on a technology – gas turbines running on biofuels – that exist only at small scale and at high cost. Furthermore,Australia’s hydro and pumped hydro facilities cannot be used in the way assumed in the simulations.
Reliability of supply
The system simulated by EDM-2011 would not provide a reliable electricity supply. The gas turbines running on biofuels and hydro-electricity provide nearly all the power, outside sun hours, on some winter days, e.g. July 1 to 6 for 2010 (Elliston et al., 2011b, Slide 12). However, the gas turbines running on biofuels system does not currently exist at commercial scale. Furthermore,Australia’s total hydro capacity cannot be run at full power for days and weeks at a time as is assumed in the simulation. As such, without the assumed generation from these two technologies, the system simulated has near zero generating capacity for many hours in winter. This would mean load shedding or rolling blackouts across theNEM, with no electricity for most consumers during those times.
If we substitute natural gas for biofuel for the gas turbines, we’d need capacity about equal to the winter peak demand (33 GW) to provide a reliable electricity supply with about 20% capacity reserve. That means, nearly all the generation would be by natural gas on some days in winter. The plants would be ‘peaker’ plants, not ‘baseload’, so they would be open cycle gas turbines (OCGT), which are the inefficient, high cost of electricity, high CO2 emissions type of gas technology.
Cost
For the baseline scenario (Scenario 1) the electricity supply would be unreliable and the costs for a system built in the current decade are estimated to be around $568 billion capital cost, $336/MWh cost of electricity and $290/tonne CO2 abatement cost (Figure 6).
That is, the wholesale cost of electricity for the simulated system would be seven times more than with the existing system, with an abatement cost that is 13 times the starting price of the Australian carbon tax (Energetix. 2011) and 30 times the European carbon price (European Energy Exchange, 2012). (The cost of electricity does not include the costs for the existing electricity grid).
For Scenario 2 (natural gas substituted for biofuel in the baseline scenario) the cost of electricity is estimated at $280/MWh (Figure 6), which is about six times the 2009-10 average cost of electricity generation in theNEM. The power supply would still be unreliable, but less so than with gas turbines running on biofuels.
For Scenario 3, where the assumptions are changed to provide a more reliable, mostly renewable electricity supply (although still not as reliable as we have now), more gas would be used and the cost of electricity is estimated at $286/MWh. CO2 abatement cost is estimated at $306/MWh (Figure 6).
Scenario 4 – If the transmission capacity is reduced the capital cost and cost of electricity are further reduced (Figure 6) but more gas is used and more CO2 emitted (Figure 5). This scenario has the lowest capital cost and lowest cost of electricity.
The assumed ‘copper-plate’ transmission system (Scenarios 1 to 3) adds $107 billion to the capital cost and $58/MWh to the LCOE (Figure 7). The reduced additions to the transmission system (Scenario 4) adds $67 billion to the capital cost and $37/MWh to the cost of electricity (see Figure 7). These costs are included in the capital costs, cost of electricity and CO2 abatements costs.
The transmission system additions are a high cost, especially when we consider there is no increase in demand driving these extra costs. These costly transmission upgrades are only required if the policy objective is to implement renewable energy, rather than to provide low emissions electricity at least cost..
Baseload
EDM-2011 conclude “Achieving 100% renewable electricity also entails a radical 21st century re-conception of an electricity supply-demand system.” They make their point succinctly in the last slide in their slide presentation where they state “Baseload plant is an outmoded concept” (Elliston et al. (2011b).
However, since the cost of electricity from the renewable energy option is some seven times the current cost of electricity, their study does not refute the fact that the “baseload plant” is still by far the least cost way to supply most of our electricity needs, and is far from being an “outmoded concept”.
The least cost way to meet the demand and reliability requirements is with a mix of generators that are located close to the demand centres, connected by relatively short transmission lines to the main demand centres and capable of supplying the power to meet baseload at all times, intermediate load during day time on week days and peak demand whenever it occurs.
The least cost option to match generation to the demand profile in most countries where large hydro capacity is not available such as inAustralia, is usually with coal, gas or nuclear for baseload, gas and hydro for intermediate load, and gas and hydro for peak load.
Bayless (2010) in “The case for baseload” provides “an engineer’s perspective on why not just any generation source will do when it comes to the system’s capacity, stability and control”. He says:
The electric system is more than just the delivery of energy—it is the provision of reliability. First, the system must have capacity, that is, the capability to furnish energy instantaneously when needed. The system also must have frequency control, retain stability, remain running under varied conditions, and have access to voltage control. Each of those essential services for reliability must come from a component on the system. Those components are not free, and they don’t just happen. They are the result of careful planning, engineering, good operating procedures, and infrastructure investment specifically targeting these items.
The simple cost analysis presented here demonstrates that the renewable electricity system simulated by EDM-2011 cannot meet these requirements at anywhere near the cost of a conventional system.
Conclusions
I have reviewed and critiqued “Simulations of Scenarios with 100% Renewable Electricity in the Australian National Electricity Market” by Elliston et al. (2011a). That paper does not analyse costs, so I have also made a crude estimate of the cost of the scenario simulated and three variants of it. I conclude:
The costs for the simulated 100% renewable electricity system are estimated to be $568 billion capital cost, $336/MWh cost of electricity and $290/tonne CO2 abatement cost. That is, electricity would cost seven times more than now, and CO2 abatement cost would exceed current carbon prices by 13 times the starting price for the Australian carbon tax and 30 times the European carbon price (at time of writing).
The electricity supply would be unreliable.
Any largely renewable electricity system for theNEMwould be high cost, as demonstrated here. The changes made to the assumptions make little difference to the estimated capital cost, cost of electricity and CO2 abatement cost.
Recommendations
I recommended the simulation be rerun with the following changes:
- Use natural gas instead of biofuel
- Increase the gas generation capacity so there is sufficient capacity in the system to meet all peak demand and ensure 20% capacity reserve.
- Check that the system can meet demand at the 5 minute time scale, not just the average demand over 1 hour.
- Introduce constraints on hydro generation, pumped hydro energy storage rate, times of day for pumping and for generating and minimum number of continuous hours of pumping that match the actual constraints on the actual plants in theNEM.
- Reduce the capacity of transmission lines from the wind farms to a percentage of their rated power output and reduce the maximum output of the wind farms accordingly; optimise (roughly) the transmission line capacity and generating capacity to achieve the least overall cost of electricity from the system.
- Limit the peak output of the PV generators at a percentage of their peak power output to fit within the constraints of the distribution system; optimise (roughly) to achieve the least overall cost of electricity from the system.
- Limit the capacity of the interstate transmission interconnectors (this would reduce the output of the renewable energy generators at some times and reduce the pumped hydro storage rate).
- Do a loss of load probability (LOLP) analysis to check that the system being simulated meets the Australian Energy Regulator’s reliability requirements.
- Do a simulation with a nuclear power scenario to provide an objective comparison of the cost for an alternative way to provide a low-emission electricity supply.
Estimate the costs of all scenarios and compare them on the basis of:
- CO2 emissions intensity
- capital cost
- cost of electricity
- CO2 abatement cost
Acknowledgements
I would like to thank Professor Barry Brook, Dr. Jani-Petri Martikainen DrJohn Morgan, DrIan Nalder, Martin Nicholson, Graham Palmer, Dr. Gene Preston, Dr. Ted Trainer and two others in the electricity industry whom I cannot name, for their input and assistance with this analysis and reviewing this document.
References
ACIL-Tasman (2010), Preparation of energy market modelling data for the Energy White Paper
http://www.aemo.com.au/planning/0400-0019.pdf
AEMO (2011), South Australian Interconnector Feasibility Study
AER(2011), State of the Energy Market 2011
http://www.accc.gov.au/content/index.phtml/itemId/1021485
Australian Natural Resources Atlas, Land Use – Australia
http://www.anra.gov.au/topics/land/landuse/index.html#lands
Bayless, B. (2010) The case for baseload
Beyond Zero Emissions (2010), Zero Carbon Australia – Stationary Energy Plan
http://media.beyondzeroemissions.org/ZCA2020_Stationary_Energy_Report_v1.pdf
DCCEE (2010), National greenhouse accounts (NGA) factors, Table 5
Diesendorf, M. (2010), Ambitious target does not measure up.
http://www.ecosmagazine.com/paper/EC10024.htm
DRET (2011a), Energy in Australia – 2011
http://www.ret.gov.au/energy/Documents/facts-stats-pubs/Energy-in-Australia-2011.pdf
DRET (2011b), Fact Sheet – Australian Electricity Generation Technology Costs – Reference Case
http://www.ret.gov.au/energy/facts/Pages/EnergyFacts.aspx
DRET (2011c), Data – Renewable Performance and Cost Summary 2011
DRET (2011d), Data – Fossil Fuel Plant Performance and Cost Summary 2011
Electropaedia, Electricity Generation with Biofuels
http://www.mpoweruk.com/biofuels.htm
Elliston, B., Diesendorf, M. and MacGill, I.(2011a), Simulations of Scenarios with 100% Renewable Electricity in the Australian National Electricity Market
http://www.ies.unsw.edu.au/docs/Solar2011-100percent.pdf
Elliston, B., Diesendorf, M. and MacGill, I.(2011b), Simulations of Scenarios with 100% Renewable Electricity in the Australian National Electricity Market. (Slide presentation)
http://www.ceem.unsw.edu.au/content/userDocs/Solar2011-slides.pdf
Energetics (2011), Carbon price impact on energy prices
http://www.energetics.com.au/newsroom/energy_newsletter/carbon-price-announcement
EPRI (2010), Australian electricity generation technology costs – Reference case 2010
http://www.ret.gov.au/energy/Documents/AEGTC%202010.pdf
European Energy Exchange (EEX) (2012), European Emission Allowances
Grattan Institute (2012), No easy choices: which way to Australia’s energy future? Technology Analysis
http://www.grattan.edu.au/publications/125_energy__no_easy_choices_detail.pdf
IEA (2007), IEA Energy Technology Essentials – Biomass for Power Generation and CHP
http://www.iea.org/techno/essentials3.pdf
IEA/OECD (2010), Projected costs of generating electricity
http://www.mit.edu/~jparsons/current%20downloads/Projected%20Costs%20of%20Electricity.pdf
Lang, P. (2010), Australia‘s pumped hydro energy storage capacity, Oz Energy Analysis
http://www.oz-energy-analysis.org/feed/show_me.php?comm=OzEA_DG0002
Nicholson, M. and Lang, P. (2010), Zero Carbon Emissions – Stationary Energy Plan – Critique
http://bravenewclimate.com/2010/08/12/zca2020-critique/
NREL (2011), Levelised Cost of Energy Calculator
http://www.nrel.gov/analysis/tech_lcoe.html
Simms, R. et al (2009) “IEA’s report on 1st to 2nd Generation Biofuel Technologies”
Trainer, F. (2010) Another ZCA 2020 Critique
http://bravenewclimate.com/2010/09/09/trainer-zca-2020-critique/
[1] Crude estimate of LCOE: PHES plant would buy renewable energy when it would otherwise be spilled and would have to sell at about 4 times the buy price for PHES to be economically viable. If we assume electricity is bought at average $75/MWh, then LCOE for generation from PHES would be 4 x $75/MWh = $300/MWh.
Filed under: Emissions, Policy, Renewables
Excellent analysis Peter.
GT’s are indeed highly flexible Brayton tech. They can run on anything liquid or gaseous. Most can be configured to run on both when either is available. They run them on crude oil in Saudi Arabia in fact. The problem is the creation of such vast amounts of biofuel to run them on. The infrastructure for such a project is only imaginable without the costs included, IMO.
Secondly, ‘storage’. There is always a few half-truths invovled with this.
Obviously a CSP plant that has 24 hour out put can only do so at about 1/6 the name plate capacity. The ability to hold usuable heat say, 8 hours after the sun goes down is quite limited and, questionable as to reliability. Additionally, it is also weather dependent. But more importantly…these massively expensive proposals are limited to just that: ONE SET OF 24 hours of declining energy reservoirs. What happens if there is a regional, say, 1 day overcast? What then? Suppose the morning fog, something we get in California or a winter storm blocks out the sun…what kind of reliability then exists for such a scenario? It is a very one.
Very informative post. Thank you. I would just add few remarks on the biofuels:
1)Low energy density which is inconsistent with required labour productivity in developed countries: I recently read a book “The biofuel delusion”, by Giampietro and Mayumi which discussed this quite well. If we have a society where kids go to school, older people retire etc . etc, then labour metabolic rate MJ/ working hour must be very high in the productive sector. These rates are powered by the energy sector where the rate must be higher still (20000-40000MJ/hour apparently in the developed world). With biofuels it will be very hard to achieve the required metabolic rates. They had a clear example on whether crop residues are suitable feed for livestock. Answer: In undeveloped world they could be, but in developed world to break even economically you need so much higher labour productivity that concentrate feed is required.
2) Many biofuel schemes are questionable from climate perspective. When fossil inputs to agriculture and the effects of land uses changes are taken into account they essentially amount to repackaging of a barrel of oil into barrel of biofuel.
3) When mankind already appropriates maybe around 30% of continental primary production and this is the main driver of extinctions, burning some more living things into energy seems crazy. It just adds to the pressure.
4) Removing crop residues should not be assumed harmless. As far as I understand, one of the reasons why African soils are so depleted is their need to use of crop residues as energy.
Great work PL . I totally concur with the above comments. And Jani, it is indeed critical in an advanced society to have available : abundant ,affordable and clean energy , 24/7. I love the labour metabolic rate you mention, it actually is good shorthand for why certain societies have higher incomes than others. Actually I would argue that a high metabolic rate is a prerequisite, if not a sine qua non, for any society to function well in the modern world.Of course nuclear is the only way to go here, as explained so well in the above post. Finally , I would like to mention again the likely objection solar concentrating plants would get from the indigenous peoples whose land it actually is where they would be built upon.
David Walters, Jani Jani-Petri Martikainen and unclepete, thank you for the informative comments. I know little about biofuel so all the contributions we can get about this will be great.
My focus, of course, is on the cost of the system.
I’d like to get a better understanding of how a biofuels electricity generation system could work as a ‘peaker’ plants that operate at just 13% capacity factor and run only for a few short periods in winter time, as illustrated by Slide 12 here: http://www.ceem.unsw.edu.au/content/userDocs/Solar2011-slides.pdf
By system I mean all the components of the biofuel production, transport, storage, distribution for biogas or for liquid fuels. How much land area would be required at worst case (in droughts), If we need irrigated land, then how much water would be required, How would the biofuels be collected and transported to the power station.
Most importantly, what would be the total cost of the biofuel delivered to the gas turbine for a system to generate 24 GW of power, in ‘peaker’ plants with an average at of 13% capacity factor and all generation over a few short periods in winter?
The metabolic rate observation seems to be an equivalent formulation of the ‘EROEI cliff’ described here. That is an advanced society needs high net yield primary energy sources. Peter Lang’s detailed calculations seem to bear this out. Biofuels are a useful niche where they accumulate as waste at places like dairy farms, fast food restaurants, landfills and sawmills. Behind the scenes diesel and coal fired electricity are doing the real work. Waste product biofuels are quite different to growing and harvesting them on a massive scale as a matter of necessity.
Household batteries coupled with smart meters may be a way to use and store widespread PV. In Australia that could mean perhaps $50k investment per house times times say 5m homes or a mere $250bn.
There seems to be no simple calculation that comes up with an optimum renewables penetration like 20% but we know it is less than 100%. We could eventually discover that number if the biases were removed from the system, perhaps subject to an overall carbon constraint.
Ah Peter Lang… When he is good, he is very very good indeed. Invaluable contribution, thank you Peter.
Peter Lang — Well done indeed. I suggest using generation curtailed rather than spilled as the later strictly only applies to hydro operation upon resevoir at capacity or to meet minimum streamflow requirements at times of low demand for electricity.
Peter, let’s hope you get more response from the EDM team than we got from BZE on the ZCA2020 critique.
This kind of in-depth analysis (as with the ZCA2020 critique) deserves a comprehensive response from the authors of the original paper. To not respond suggests an irresponsible disregard for the potential value in the critique. I trust you will make sure that the EDM team get a copy of your paper.
This is a huge and truly impressive piece of work. Peter is to be congratulated for the effort he’s put into this and the detail of his analysis.
The discussion of each of the component technologies is particularly valuable, and could stand alone, without reference to the EDM paper. If anyone is finding the sheer size of this paper offputting, I’d suggest reading the “Comments on the technologies and assumptions” section.
It demonstrates that one cannot simply abstract a high level representation of these generators and bolt them together to make an energy system. Rather, one has to deal with the messier physical realities of the dynamics and latencies, costs and scaleabilty, spatial distributions and temporal variabilities, and not least the economics. To borrow a term from philosophy of mind, it is their qualia that matters, and that many of the renewable plans ignore.
The biogas discussion in particular brought this home for me. The substitution of biogas for natural gas in discussion of backup power looks like a sleight of hand – they aren’t substituteable, as Peter lays out in these points:
– the existing biogas capacity is not substantially gas turbines but reciprocating engines – steam power, or perhaps internal combustion. Small, local, and with very different characteristics to a gas turbine.
– The biomass required to cover the winter gap in solar amounts to ~74% of Australia’s irrigated land.
– Supplying a biogas plant would require a ~1-200 truck loads every day from about ~100 km catchment.
– The latency between biomass collection to biogas production is of the order of a month. So like other technologies the biogas plant capacity needs to be “overbuilt” so stockpiling feedstock and product biogas can begin ahead of the winter demand.
– Large biogas storage tank capacity must be available at the biogas generator sites, sufficient to cover a large chunk of national energy demand through the winter weeks.
The cost of this would be enormous, even if it weren’t practically impossible, for infrastructure that is mostly to be used in a single season.
High level descriptions of systems are easy; detail is hard. The inclusion of a large biogas component in the EDM work suggests a cavalier disregard for detail, and therein hides the devil.
Peter, excellent analysis.
Like JN and JM, I think the biofuel proposal is hugely problematic.
Kessides and Wade have an interesting measure of dynamic EROI, which permits a more nuanced measure of EROI. They make the point in regards to wind (although the same applies to biofuels), that despite possessing a similar static EROI to coal, the ability of wind to rapidly scale up its production by
bootstrapping its own energy appears to be far more limited. I suspect this is what most of us suspect anyway – are wind, biofuels, etc going to be able to generate enough surplus energy within ecological limits to run the mines, processing, factories, transport, homes, agriculture etc.. that is required in a modern industrial society?
http://www.mdpi.com/2071-1050/3/12/2339/pdf
I agree with Elliston et al. that there are many potential opportunities to exploit agricultural wastes (and other wastes), but the whole bioenergy discussion is a huge can of worms, ecologically, economically and logistically.
[…] answer whether a system of 100% renewables can meet Australia’s 2011 electricity demand. Detailed critique and analysis shows that it cannot. Costing done independent of the study itself (… For the EDM-2011 baseline simulation, and using costs derived for the Federal Department of […]
DBB, thank you for that suggestion. You are correct that “spilled is not a good term, but it is widely used and is used throughout the paper I critiqued.
There is a better term that is commonly used, but it has slipped my mind for now. I’m sure I’ll remember it as soon as I post this comment.
Martin Nicholson: good points. Thank you. Yes, I will send the authors a copy of the PDF. And thank you for your help.
John Morgan thank you for your comments, and for all your help too. I suspect your comments also apply to liquid biofuelled gas turbines.
Graham Palmer, thank you for your interesting comments and excellent help too. I hope you will expand on this as the thread progresses.
Peter Lang — NREL uses curtailed.
Peter, well done.
It is a nice critique, the cost analyses in particular are excellent. I really hope they respond (unlike BZE!) so we get some constructive discussion happening between BNC and Elliston, et al, and hopefully identify some common ground.
I have some extra points to add to the PV section, I’ll get back to you soon.
Cheers
Although only briefly mentioned in both the Elliston paper and critique, the issue of peak demand reduction is critical in a reducing the need for infrequently used capacity. The Elliston paper, the BZE report, and nearly every pro-renewables proposal assumes that energy efficiency reduces both consumption, and peak demand ipso facto yet the literature on the relationship between efficiency and peak demand is actually quite sparse.
Putting aside Jevon’s Paradox, perhaps the greatest challenge in these Australian plans is providing sufficient capacity for Melbourne during winter. Forget smart grids, TOU pricing, and extra insulation – on a near freezing morning or evening, households want to press the thermostat and get warm. This is an essential service that must be provided reliably and affordably for all households as an issue of social justice. The key issue is that when people get home from work and turn on their heater, and it runs at full capacity for an hour, it doesn’t matter whether extra insulation reduces the annual energy consumption, the peak demand remains. Ironically, leaving the heater on all day will result in reduced contribution to aggregate peak demand during the “peak hour”, but consume more energy. It can be shown that energy efficiency can provide some aggregate reduction in peak demand, but the relationship is by no means simple, and usually limited.
In this regard, the Elliston paper is far more sensible than the BZE report, which advocates shutting down the natural gas network. The large scale conversion to electric heat pump would worsen peak demand due to the need to run a defrost cycle on the coldest days, and operate at substantially reduced efficiency at low ambient temperatures.
Brilliant work, Peter.
Barry, thanks for bringing this to us.
Care to tell us why this is the case. I don’t see where the authors have widely missed the mark on hydro storage capacity and requirements. 2.2 GW capacity at 20 GWh DOES reflect an upper storage limit … it’s roughly 9.1 hours of continuous pumping (before the max is reached). Water availability is not factored into their model. I also don’t see where they intend to use stored energy during “most of the year” for release during a “few short periods in winter.” As they state in the paper, PHES is utilized for storing “spilled” energy and daily balancing to maximize yearly gross energy production: “In this scenario, pumped storage hydro plants are charged using spilled energy and dispatched first to maximize the energy supplied year round” (p. 3). They state the main benefit from hydro storage (at the low energy storage levels achievable in Australia) is not meeting peak demand, but offsetting biogas consumption (p. 9).
Your other recommendations are spot on: it would be great to have a run of their model on 5 minute scale, LOLP analysis (rather than statistical comparison with 2010 load profiles), and addition of a non-solar dependent 24 hour baseload variable (geothermal, nuclear, CCS) and look at how this changes operational criteria (with respect to spillage, gas utilization, storage demands, unmet demand, etc.). Since there is a great deal that they are not doing in this study yet (Slide 7: providing a plan, covering all regions, covering all end-use, demand response analysis, transmission modeling, economics), it sounds like NOW is a pretty good time to have a constructive impact on the full shape and scope of this long-term research project (for this conference paper, and in the future).
Graham Palmer,
Thank you. Excellent points.
I’d add, if we want to reduce emissions from all energy consumption, electricity will have to replace gas for heating. That means the peak Melbourne cold morning peak demand would be even higher.
John Bennetts, thank you for the comment. Can you suggest any refinements for the transmission estimate (see Appendix 2 in the PDF version)?
EL,
Thank you for your questions and comment. I’ll answer your questions in two separate replies.
I need to provide more explanation on this. I’ll give a short answer here and intend to write up a more detailed comment to post on the Pumped Hydro thread and perhaps on OzEA to add to the previous comment here http://www.oz-energy-analysis.org/feed/show_me.php?comm=OzEA_DG0002 which addressed the question:
EDM-2011 cited this comment on OzEA as the basis of their pumped hydro energy storage capacity, but they did not realise there is a constraint on the rate at which energy can be stored.
I need to add to the OzEA comment an explanation of the rate at which energy can be stored in the three existing pumped hydro plants.
Is the short answer:
“20 GWh DOES reflect an upper storage limit”. This satatement is correct.
“2.2 GW capacity”. No. That is not correct. Wivenhoe is the only pure pumped hydro scheme in Australia. The other two PHES plants have some pumped hydro capacity within a plant that is mainly a hydro plant. EDM-2011 attributed the full generating capacity of these plants to pumped hydro.
Only 0.9 GW of generating capacity can be attibuted to pumped hydro. The remainder of the 2.2 GW EDM-2011 assumed should be attributed to hydro. Furthermore, there are energy losses in pumping and generating, so only about 75% to 80% the energy can be recovered.
Wivenhoe PHES:
All the water Wivenhoe PHES plant uses to generate power must be pumped up from Wivenhoe reservoir. It can store energy (that is energy that can be recovered, after losses) at the rate of about 328 MWh per hour. It pumps when demand is low and electricity is cheap – between about midnight and 6 am (longer on weekends). It is on standby (spinning and ready to go to full power within less than 1 minute) for about 12 h per day and generating to meet peak and intermediate demand for about 7 hours per day (at variable power output). It generates a little during standby to balance power and frequency fluctuations in the grid. It cannot pump while it is generating or on standby. Because it is needed to be generating and on standby during the day, and because power is cheap at night, the pumping is done at night when the plant is unlikely to be needed for generation.
It takes a lot of energy to start pumping the water. The weight (~23,000 tonnes) of water must be lifted up 100 m against gravity and accelerated from 0 to 3.6 m/s velocity each time pumping starts. Therefore, it is not economic to repeatedly stop and start the pumping. The pumps are not variable speed so once started they run at their constant pumping rate of 207 m3/s.
Therefore, the pumps should only be started if they can be assured they will pump for at least several hours (e.g. 5 hours, but I said let’s assume a minimum of 4 hours to be economically viable).
If we assume an average of 5 hours of pumping time per day and energy storage at the rate of 328 MWh per h, then Wivenhoe can store about 1,640 MWh per day.
Tumut 3 has 1,500 MW of generating capacity in six generating units but only three have pumps and the power of the pumps is only little more than half the power of the generators. So it is not appropriate to assign the 1,500 MW of Tumut 3 generating capacity to PHES.
Bendeela & Kangaroo Valley – similar comment to Tumut 3.
See more details here:
http://www.oz-energy-analysis.org/feed/show_me.php?comm=OzEA_DG0002
EL @ 10 February 2012 at 7:21 AM
Part 2 to my reply to your questions
But EDM-2011 also point out the system they simulated cannot meet the demand on some days in winter. EDM’s figure 2 does not make the problem clear because the 0 to 2.2 GW on the vertical axis has been truncated. This is where the pumped hydro should be displayed. So, I refer readers to their Slide 12 here: http://www.ceem.unsw.edu.au/content/userDocs/Solar2011-slides.pdf.
The dispatch order has been changed so pumped hydro is not the first to be dispatched. The dispatch order is:
• Wind,
• PV,
• CST,
• pumped hydro,
• hydro,
• gas turbines running on biofuel.
This is a more sensible and realistic dispatch order, than the one presented in the paper, from the point of view of trying to minimise the cost of electricity (given the technologies assumed by EDM-2011, their capacities and capacity factors).
Slide 12 shows that very little pmped hydro is available during the critical days in winter. For the 8 days shown in Fighre 12, there is no pumped hydro generation on four days, and very little on the other four. In fact, the the CST plants have not even been able to recharge their storage on those days.
My point was that the pmped hydro is most needed on those days in winter. To be most effective, pumped hydro would need to be maitained at full storage before entering those periods in winter. That is storage throughout the year until those critical periods in winter. This is what is critical for reducing the total capacity of biofueled gas turbines that would be required to provide a reliable electricity supply. The use of pumped hydro to reduce the amount of biofuel energy generated is a seaparate issue from the peak generating capacity required.
Just to repeat the point of your comment/question:
The point I was attempting to make is that pumped hydro is of little value in helping to meet winter peak demand. It also plays only a small role in summer at huge cost to avoid a little generation from biofuels (from looking at Slide 11).
I hope this has addressed this comment/question satisfactorily.
Just to head off any tendency to get down into a pedantic discussion about points of figures that have no significant bearing on the costs, can I suggest that we focus discussion on issues that do have a significant bearing on costs.
Sure enough, charge rate does appear to have a bottleneck at existing pumped hydro facilities (and other hydro plants) in Australia. If they intend to continue to use existing facilities in their calculations, they will have to update their figures in their model, and adjust for any efficiency losses for shorter term charging cycles. Perhaps this is what they meant when ruling out “water availability” in their calculations … excluding both environmental resource constraints and charge rates on existing facilities (up to full available capacity). It should be helpful to have these numbers published and documented here and elsewhere.
As concerns feasibility and costs of PHES in their paper, I’m not sure why they limit their analysis to “existing hydro” when looking at energy storage options for NEM? They are clearly proposing a broad buildout of generating technologies (CST and more), why not do the same for energy storage and run their model to more fully cover the winter period, maximize capture of spilled energy, and use less biogas on an annual basis? In Australia, energy storage from pumped sea water should be an option (anybody else know about specific proposals). Japan has an operating plant (31 MW with about 6 hours of storage), and Ireland is looking to do the same (980 MW with some 6 hours of storage). A Diurnal Storage Presentation (p. 14) at a recent DOE energy storage workshop suggests ocean pumped and variable speed PHES is less than five years from commercialization (with stainless steel components and fiber-reinforced plastic tubes in penstock and tailrace to minimize corrosion and barnacle concerns). A peer reviewed study from 2010 suggests CAES as a more affordable option than PHES for Australia, with an estimated 15.4% rate of return on capital investment (as compared to 9.6% for a sea water pumped hydro plant). Natural caverns, old mines, depleted gas, and aquifer reservoirs may be available for larger CAES facilities. Obviously, with lower cost fossil fuels available for capacity reserves there’s not much economic incentive to currently pursue these more expensive storage options (unless rising fuel prices, high marginal costs, supply interruptions, policy mandates, carbon costs, technology breakthroughs, or other externalized costs of fossil fuel generation are added to the picture).
One other issue concerning cost. Traditionally, PHES has been a strategic tool for baseload developers, especially in helping with thermal power efficiency and flattening out load variations. So while expensive, it often has operational and power utilization benefits that far outweigh it’s per kWh levelized investment cost. Increasingly, markets are starting to reward these benefits, and so comparing this to the wholesale rate of energy may not be particularly illuminating. How to properly price the value of stored energy (for such thing as distributed generation and power quality end-use, ramp rate control and load time shifting, voltage regulation and capacity services, transmission stability, and anything else) is anybody’s guess at this point. At the same DOE conference mentioned above, the lead presentation (p.49-51, and throughout) mentions a list of proposals for market reform at national level, state, and among PUCs in the US to perhaps make better use of ancillary and energy storage services (apart from generation). Perhaps something like a rate of return on investment might be a better measure for understanding the comparative costs of storage plants (rather than levelized costs of the energy that is being produced at them)?
EL,
Thank you for your comment. You say:
It is hydro, not pumped hydro, that is limited by the water inflows to the storage reservoirs. So this part of your quote is not relevant to the discussion about pumped hydro. I think EDM have, in fact, limited their total hydro generating capacity (Hydro + PHES) to about what the NEM generated from hydro in 2010. The problem I highlightred was that their allocation of generating capacity between hydro and pumped hydro is not justified (IMO).
It is the figure of 2.2 GW of pumped hydro generating capacity that is the misleading figure they have used (IMO). I’d suggest they shlould use 0.9 GW of PHES generating capacity because that is the sustainable maximum. The average would be much less than this (if charged with coal or nuclear) and much, much less if charged with intermittent renewables).
I’d give two reasons:
1. Because the Greens and environmental NGO’s and their followers are almost as strongly opposed to hydro ans they are to nuclear (not just in Australia but in other developed counmtries too.
2. Pumped hydro is not viable unless you have low cost baseload power supply – like brown coal or nuclear (if we would allow low cost nuclear).
If you would like to get some background on the viability of pumped hydro in Australia could I refer you to this BNC post and in particular to the two reviewers comments included near the end of the article.
http://bravenewclimate.com/2010/04/05/pumped-hydro-system-cost/
Also, you will find many excellent posts in the comments following that article.
EL. That is an excellent idea. Why don’t you set an example for other BNC readers. Shw them how easy it is. Open the spreadsheet linked at the top of the thread, then change the input parameters and record the change in:
1. Capital cost for the whole system
2. Cost of electricity for the whole system (excluding the cost of electricity for the existing NEM grid network
3. CO2 abatement cost for the whole system
You could produce a chart showing the cost of your optins and link it for others to see here.
The inputs you’d need to change are:
1. the generating capacity for PHES (You’d need to change the capacity factor and perhaps the generating capacity for OCGT (i.e. gas turbines running on biofuels) to keep the total electricity generated at 204,400 GWh.
2. the interstate transmisison system capacity (to carry more power from the renewable generators to pump the water)
3. increase the 204,400 GWh of total generation to account for the additional transmission losses and losses in pumping.
I’d strongly urge you to backgropund yourself on the pumped hydro thread. Large man-made salt lakes at altitide are not likely to be a popular idea. This thread will give you some idea of the size of the lakes that would be required to power Australia with solar and pumped hydro alone (scale appropriately to answer your questions):
http://bravenewclimate.com/2009/08/16/solar-power-realities-supply-demand-storage-and-costs/
This should be enough to get you started in being able to answer some of your own questions. You will gain a lot by having a go at doing some simple number crunching yourself. You will learn a lot more than simply asking masses of questions. I presume you have read David Mackay’s excllent book “Sustainable Energy – without the hot air”?. http://www.withouthotair.com/
You’d recall his main purpose for writing the book was:
http://www.inference.phy.cam.ac.uk/withouthotair/c0/preface.shtml
An interesting anecdote: I had a chat to an engineer who was ex-Southern Hydro about the recently built Bogong Power Station (which was built by – apparently they were designing it so that they could fit it out for pumped storage as an option
All they was needed was to dig another tunnel during, which isn’t too expensive if you already have a TBM on site compared to retrofitting it later.
It seems they were turned off the idea when the government said that they wouldn’t be able to get as many Renewable Energy Certificates if they pumped water using power from the grid, due to the Latrobe Valley coal power plants providing most of said power. It seems that grid energy storage might be a bit disincentivised unless it’s actually bolted onto a wind farm or solar power station.
Something tells me that back in the days of the SECV that they would’ve built it as pumped hydro anyway – the Victorian grid used to be rather inflexible and they had a few scares with that in the late 70s/early 80s, causing a dash for gas-fired peakers.
New map of transmission lines added to the top of the post.
8 hours of storage at 20% of peak demand should be entirely sufficient for what we are talking about here. It’s certainly a great deal more (by a factor of 2.6) than what they are drawing on in the EDM-2011 study. For good measure, let’s double the amount to 40% for any projected long-term increases in demand. I don’t find the geographic dimensions of this storage amount to be all that daunting. In previous threads, I have used the Ludington Storage Plant in Michigan as a dimensional reference point.
– NEM peak energy demand = 33 GW (2007-08).
– 40% peak demand (at 8 hours): 13.2 GW capacity (at 105.6 GWh energy storage)
– Ludington PHES dimensions and cost: 1.872 GW capacity, 14.976 GWh energy storage, 3.4 square km surface area (built on top of a sand dune beside Lake Michigan with no bedrock foundation), $327 million in 1969 (some $2 billion today, or in today’s cost $133/kWh). An $800 million upgrade announced in 2011 will extend the total operational lifetime of the plant to 84 years, and boost capacity to 2.172 GW (at an additional cost of $333/kWh). Annual O&M is $11.6 million/year. I wish I had yearly storage data for the plant, might be an interesting exercise (especially looking at full 84 year operational lifetime).
– Equivalent size of seawater reservoir to meet NEM 40% of peak demand for 8 hours: 23.97 square km (or 5923.12 acres). This is roughly 1.5 times the area size of the Lake St. Clair Reservoir (Glennies Creek Dam) near Barrington Tops National Park in New South Wales. Cost at USD $300/kWh = $31 billion. As previously stated, energy storage is increasingly being valued separately from generation resources. The study I cited above indicates a possible rate of return of around 9.6% for seawater PHES in Australia.
Only in the worst of possible straw man arguments does anyone suggest storage amounts for an entire grid in the range of 3 to 10 to 30 days (or more). No such thing is contemplated by the EDM-2011 model, and I know of no single peer reviewed study that would suggest the same. It’s simply not how our energy system works (or is conceived to work on a cost-effective basis in the future). Resource planning, transmission design, demand management, and capacity reserves are fully adequate to the task of meeting seasonal shifts and shortfalls, and as the EDM-2011 model attempts to show (perhaps with greater storage levels suggested above), much of this can be supplied with carbon-neutral (or very low carbon) alternatives. And for the environmentalists alarmed over freshwater resources and new hydro development, no natural rivers or lakes were harmed in the making of this saltwater PHES storage portfolio!
@EL: If you think that asking for 3 days+ storage is a strawman argument, how do you explain the EDM-2011 Figure 2? The huge amount of biofuel and hydro *is* long term storage. Just in the form of biological sunlight->chemical fuel conversion, rather than vast amounts of pumped hydro. It is also, as noted, not very plausible, in a similar way to replacing it with more solar+long term pumped hydro storage isn’t very plausible.
EL,
You didn’t say if you had read the two threads I provided the links to.
I’d urge you again to background yourself on the two threads I linked that have already been written on pumped hydro.
Then use the spreadsheet linked at the top of this thread and do the cost calculations so you can see how much difference you can make tot the overall system costs by increasing PHES capacity. Include the other factors that affect the costs I mentioned in my previous comment – like additional transmission capacity.
There is no point me chasing around all your ideas and references if you are not prepared to get up to speed on what has already been done. Otherwise, I’d just keep explaining what has already been said before. I’ve done that repeatedly in the post for others, and they still kept repeating their misunderstandings and wrong assumptions – over and over again. So I am not prepared to go down that route again.
You also need to do the cost calculations You need to do those yourself. Without them the discussion is just “twaddle”.
Factors you need to consider when talking about pumped hydro are where can you find the valleys that are vertically separated by say 100 to 200 m (from minimum operating level in the upper reservoir to full supply level in the lower reservoir), and horizontally separated by a short distance (e.g. 420 m for Wivenhoe, 500 m for Tumut 3).
Then you need to do the financial calculations to see if it is viable (as per the reviewers comment in the pumped hydro post).
Regarding sea water, where are you suggesting we make the upper reservoir? How high above sea level will it be when it is at minimum operating level? Is there a problem with the sea water percolating into the ground water? If you are going to suggest lining the reservoir, how and at what cost? Where has it been done in practice and what is the area of the reservoir?
For me to be interested in spending time following through your assumptions and your calculations, please go through what I’ve suggested and do the costings. Without the costings, I am not interested. Because I know, without doing the analyses, that it is all pie in the sky, totally uneconomic ideas.
Thanks Barry. Good map. It shows:
– there are no large capacity transmission lines to any of the proposed solar thermal sites nominated by EDM-2011, and
– the best solar areas are far removed from the demand centres and from the existing transmission infrastructure.
EL,
Put simply, would your proposal for increased pumped hydro capacity reduce the costs of electricity compared with the EDM-2011 scenario?
If not, or if you haven’t checked this out for yourself before proposing it, then why propose it?
To cost it you will need to nominate a site or sites, and estimate the key parameters needed for estimating it. You can use the Tanatangara Blowering Pumped Hydro thread as a template for costing your nominated site.
http://bravenewclimate.com/2010/04/05/pumped-hydro-system-cost/
Peter Lang will enjoy this opinion piece from Ross Cameron in yesterday’s Sydney Morning Herald, which deals directly with the topic at hand:
Good intentions but clean energy price too high
Cameron writes,
Cameron catalogues various disappointments and failures, including the crash of the Solar Flagships programme:
The main story here is the growing awareness amongst policymakers in Australia and elsewhere of “the wide gap between rhetoric and reality when it comes to clean energy”, and the cost of the clean energy technologies, a point Peter has been hammering here for some time.
Peter Lang some answers to your questions about pumped seawater hydro are here in the Seligman proposals. Without re-reading the document my recollection is for an octagonal plastic lined tank 7km across and 20m deep. It would be partly set into the cliff top 100m above the sea with the pumps and generators at the base of the cliffs. The pipes would run up the cliff face in a larger version of this Japanese prototype .
John Morgan,
Thank you for this. Your posts invariably cut to the very heart of the real issue.
I agree. I think the message is starting to get to policy makers. Actually, I think it is starting to get to the public. I think the many policy makers have known all along but, as Hon Andrew Robb, MP says, they have to manage expectations; they cannot get out too far in front of the public – as was demonstrated at the 2007 election when the Coalition, again, put nuclear energy firmly on the agenda. The public was not ready. This is why I advocate the first thing the government should do is to focus on education, as I said in this comment a few days ago:
http://bravenewclimate.com/2012/02/04/open-thread-21/#comment-149965
John Newlands, pumped hydro contributes 2% of the energy and 2% of the cost of electricity in the EDM-2011 simulation. This is not the place to focus attention – unless you can show that by doing so there could be a significant reduction to the cost of the system. Unless you and EL are prepared to do some number crunching to demonstrate a potential significant cost saving, I am left with the opinion that adding PHES capacity (at high cost) not reduce costs and this is not the place to focus attention. There is a spreadsheet linked at the top of this thread you can use to do sensitivity analyses to test your ideas.
The greatest uncertainties in the cost of electricity (proportion of total LCOE in brackets), and therefore the place to focus attention are:
– the solar thermal plants (34%)
– gas turbines running on biofuels (23%)
– transmission and distribution systems (17%
Another important point readers might want to consider is the reliability of supply from the renewable energy system. Two points not addressed in the paper are:
1. What happens when there is a large dust storm like we had starting 23 September 2009:
http://wattsupwiththat.com/2009/09/23/the-australian-dust-storm-from-space/
http://jennifermarohasy.com/2009/09/dust-storm-hits-central-eastern-australia/
It seems such dust storms are not infrequent, and the 2009 one was not unusual and not the worst.
Some questions to consider:
– How long would it take to clean the reflectors after such a storm?
– Where would the workers live while cleaning is in progress?
– How long would it take to get them to and from their accommodation to the work sites each day?
– How many accidents would be involved with the cleaning?
– By how much would the solar power stations’ output be curtailed?
– How much over build (excess capacity) would we need to ensure we had sufficient output to meet demand at all times and maintain reliable electricity supply?
– What would be the total cost of cleaning?
2. The second point on reliability of the renewable electricity supply relates to the reliability of the long transmissions lines. The following was sent to me by email a day or so ago:
Because it specifically addresses the questions you raise about the feasibility of PHES plants in Australia in lead article and in your comments (and other limitations you have raised about the EDM-2011 model). I have already answered most of your other questions. The Ludington plant (a model plant for many PHES developers) has received a great deal of public attention: by national and local sections of American Society of Engineers, and named one of 10 outstanding engineering achievements in Michigan over last 150 years. Plant is build on top of a sand dune, no bedrock supporting it, lined with asphalt and clay to prevent seepage, dimensions and costs have been provided, penstocks to reservoir rise 363 feet (or 111 meters), etc. This is a mature technology, and back of the envelope costs for PHES in Australia (along the lines of what you have provided) are not an issue. What is less well known are the engineering specifications, costs, and operational experience with saltwater. Which is why I asked if anybody has further details on this, and any specific proposals being considered in Australia. PHES storage plants with saltwater currently exist in Japan (see specifications), and are being proposed for Ireland (at a more practical 980 MW). If you have evidence that these environmental conditions can’t be met in Australia, I certainly would like to hear it.
If you wish to limit the discussion to cost factors alone (as you suggest above), then you should stop bringing up feasibility as a concern, or separate out your analysis into two separate threads on the EDM-2011 model.
Glad to see you have a sense of humor about this (even if it falls on the nerdy side of the spectrum).
EL,
No, you haven’t answered any of the questions, and seem to have ignored all I’ve said in my previous responses to you.
I’ll put the main question another way:
By how much would your proposal change the cost of electricity for the EDM-2011 baseline scenario? Answer in $/MWh together with basis of estimate.
I admit it will probably take a fair bit of work to reconcile how Seligman comes up with 6c per kwh storage cost. I recall someone suggested a pumped seawater storage trial on Army land near Wollongong. That should test reliability, leakage etc. It should have at least 1 Gwh capacity which I think is about 3.7 ML at 100m elevation. Not a waste of money considering the aluminium smelters will probably get hundreds of millions of dollars from carbon tax revenue as a salve.
John Newlands, it is a waste of money if the costs exceed the benefits. Why would you argue this pet scheme is not a waste of money but some other pet scheme (such as solar thermal, is a waste of money? On what basis are you making your selection?
The first step is to crunch the numbers. Otherwise, the discussion is simply “twaddle”.
Where is the site? How high above sea level at minimum operating level? How far from the coast? How much active water storage capacity? How will the reservoir be sealed?
Can you show us the site location on a Google Earth map?
I have gone through your spreadsheet of costs and find some of them to be really high.
For instance you have set the capital cost of CSP with 15 hours storage to be $13 363/kw. This is at least double what other studies show. In this quite recent review at Ref 1 Appendix IV the capital cost of a power tower with storage is $6146.00/kW in 2010. Power tower are going to be more expensive than well proven troughs. In your cost breakdown you start with $5109/kW as a base. This is from your source however I think that it is rather more than what the industry has seen. This study (ref 2) “The CRS Report for Congress 2008″ in CRS-89 the average cost of CSP is $3400/kW and is based on plants projected to be operating now. Even allowing for inflation your figure is at least $1000 to high
Your growth in cost to 15 hours storage is also extremely large. Here is the wikipedia entry for Andasol-1, a trough type plant with 7.5 hours storage. (Ref 3). In it details that about 13% of the final cost is the storage. So the storage cost roughly 50 million of the USD$380 million cost. The mirror field is about 58% of the cost (ref 3 table 4.3) so it cost 220 million. Doubling the storage gives a total cost of $430 million. The solar field does not have to be doubled as it is already over sized – 1.5 times would be sufficient – so it would cost 330 million an increase of 110 million. The total cost of 15 hours storage would be roughly USD$540 million – a cost per kW of $10 000/kW. This is of course for the first plant. Ref 1 has learning rates that can be justified so this cost after many units were built could well drop to $7000/kW.
Additionally you add a huge sum for transmission lines. Remember that this study is a “what-if” study and not meant to be implemented. It has large CSP centres in remote areas. However Ref 1 has this to say:
“For Australia, as can be seen from Figure 37, the DNI over most of Australia is over 2000
kWh/m2/year (5.48 kWh/m2/day average). There are many areas in central NSW, central QLD,
South Australia and Western Australia with average DNI around 2400, whilst still in close proximity
to the existing electricity grid. Therefore for the purposes of the solar thermal power analysis in this
report, all calculations have been done on the basis of DNI = 2400 kWh/m2/year.”
In a real CSP solution there would be a scattering of 50MW to 200MW units all over the states as Australia has such good solar resources. All or most of the these could be co-fired with whatever was available. I am a little bemused that the authors did not include co-firing. This has been done with CSP plants since the 80s and would eliminate a lot of the gas turbines. Moving the CSP plants nearer demand also eliminates all the hugely expensive transmission lines that you have included. Co-locating the CSP plants also has another benefit as depending on the local fuel supply the CSP plants could also not have so much storage. Co-Firing is a lot cheaper than storage and local economic decisions would made on the availability of fuel for co-firing. For that reason I have made the figure for CSP plants $7000/kW as this would reflect a mix of no-storage, 7 hours storage and 15 hours storage.
In your spreadsheet you have the incredible figure of $5000/kW for the OCGT. In Ref 4, Table ES 2, the cost for OCGT in 2010 is listed as $985/kW. Your figure is five times this. You say that this is because there are no commercial turbines operating on biofuels. However my first google hit was Ref 5 that states this:
“GE engineers have a deep understanding of syngas properties gained from 20 years’ experience and more than two million hours of GE turbines operating on low BTU fuels, including syngas.”
This does not sound like that they have no experience of running a turbine on syngas which is the output of a biomass gasifier. The gasifier is the tricky part however companies like Seimens at Ref 5 have been doing it for many years on commercial scales:
“the technology with its unique cooling screen design and the efficient dry-feeding system is based on more than 30 years of continuous product development.”
I do not think that this would mean the biofuell gas turbines would be 5 times more expensive than normal OCGT, more likely twice as expensive. IGCC coal plants are proven on large scales and the only difference would be the feedstock.
Putting these figures into your spreadsheet gives an total capital cost of 334 billion and a total LCOE of $20/MWhr. Co-firing the CSP plants would result in far less gas turbines though I have not modelled this so the figure could come down considerably if this was done as the OCGT have the highest LCOE due to the low CF.
It is very obvious from this study however that with a couple of Korean nukes in the high demand areas belting out power 24X7 at quite low cost as nukes do best most of the expensive gas turbines would go away and most of the CSP plants could revert to 7 hours storage resulting in far lower costs. For our society at present that demands 24X7 electricity this would be probably the optimum solution for the lower cost. I am going to try to get a copy of the code and if I find the time model a couple of scenarios. I would urge you to do the same.
Ref 1 – http://www.garnautreview.org.au/update-2011/commissioned-work/renewable-energy-technology-cost-review.pdf
Ref 2 – http://www.fas.org/sgp/crs/misc/RL34746.pdf
Ref 3 – http://www.nrel.gov/docs/fy04osti/34440.pdf
Ref 4 – http://www.qca.org.au/files/ER-NEP910-ACIL-BRCI0910-FinalReport-1008.PDF
Ref 4 – http://www.ge-energy.com/products_and_services/products/gasification/syngas_turbine.jsp
Ref 5 – http://www.energy.siemens.com/br/en/power-generation/fuel-gasifier/
Peter Lang,
Costing the EDM 2011 base model seems premature. The authors make it clear that the study is very much work in progress to look at some of the potential problems of “only” replacing the FF input used for electricity production in SE Australia.
I see three major problems in the base model:
1) relying on 65% of the energy generated from solar leading to a large winter deficit. This could be reduced by siting more solar capacity in northern Australia.
2) scaling up present wind farms by x13 without considering the benefits of wider geographic spread especially into Queensland and Western Australia.
3) not considering modifications to existing hydro and pumped hydro to dramatically increase short term generating and pumping capacity and expanding long term pumped hydro storage.
The base model as described seems to require an unacceptably large reliance on biomass use(13% total energy) and high peaking capacity(24GW).
To expand on the last point, many existing dams could add additional generators to increase short term hydro generating capacity(cost approx $60/kW). Tumut 3 pumping capacity could be easily doubled by replacing 3 non-pumping generators with double wound generators/pumps capable of handing variable pumping rates. You have discussed a possible Tantangara/Blowing pumped hydro scheme that would give X7 present pumping capacity(6GW) and X25 present pumped storage capacity(500GWh), which would allow a much higher proportion of wind energy to be used with lower “spill” losses .
Neil Howes and Ender,
I’ve been waiting with bated breath for you two guys to show up. I was wondering why you two had been quiet for so long. 🙂
OK. Now I have some work to do to see what you’ve found wrong with my estimates.
The reality is that a large amount of the carbon tax revenue will be misspent. If some of it goes on energy storage experiments giving baseline info that takes us forward somewhat, rather than being frittered away. Even if load following NPP is commonplace by mid-century there may be hundreds of wind farms with NG too valuable to still be used for backup. I’m not seeing evidence for the required 80% reduction in levelised battery costs.
Admittedly the best site in Australia for pumped seawater is nowhere near major transmission, the 250m high http://en.wikipedia.org/wiki/Zuytdorp_Cliffs
The Illawarra escarpment http://en.wikipedia.org/wiki/Stanwell_Park,_New_South_Wales
seems to be parkland or high priced real estate. Waitpinga Cliffs near Adelaide is also a nature reserve.
What if non-fossil demand matching electricity supply cannot be done?
@ Ender, re the high cost of OCGT, if you go through the report, I think you’ll find that this cost also includes the bioenergy processing, production, and storage plant. The current costs of OCGT reflect the fact that OCGT uses a commodity fuel (natural gas) already in large supply, and only needs the pipeline infrastructure to access it. However the bioenergy proposal requires dedicated infrastructure for feedstock supply, transport, conversion and storage over a full year, but will only be utilised for short periods during winter. See page 5 and 13 of the pdf.
Neil Howes, @ 12 February 2012 at 2:50 PM
I don’t agree any costing is premature. All the scenarios should be costed always, IMO. Cost should be the principal criteria for comparison between scenarios.
The fact that I have done a crude cost estimate without even having the output data, shows it is not hard. The researchers should be able to do the job much better than I can. The fact they haven’t demonstrates their priorities are in the wrong place.
The fact that the estimated cost of electricity (not even including the cost of the existing grid network, which must be added) is more than seven times the current cost of electricity, is thirteen times the starting price of the CO2 tax and thirty times the European carbon price, demonstrates clearly that the costs of renewables would be far too high to be viable. Such a gap could not be closed without some major technological breakthrough. And there is no sign of that happening.
Therefore, if the researchers were objective, they would have done an objective comparison with a nuclear scenario right from the start, or at least, as soon as they realised that the costs for renewables is so high they will never be viable.
You can test the cost of your idea using the Excel file. You’ll need to increase the length of the transmission lines, and the capital cost unit rate and O&M costs for CST (for the remote areas. Trades persons in mining areas are now paid about $200,000 p.a.).
Wider dispersion within the region covered by the existing wind farms will make little difference. There is high correlation within that region. Further north and inland doesn’t have good wind resources as the recently released Grattan Institute study shows. The cost of transmission connection to WA would be very high as was shown with the ZCA2020 study, stated in the Gattan Institute report and admitted in the EDM paper.
We must have discussed this a dozen times. I’ve refuted your hopes and beliefs on this every time. Refer to comments on the Tantangara-Blowering Pumped Hydro thread to remind yourself.
If you have an alternative, then state the site, provide the relevant details, estimate the cost and provide the basis of your estimate. I’ve done it repeatedly and each time shown that pumped hydro at the scale needed is totally uneconomic for renewables. There is not a hope in Hades of it being economic (at the scale required).
That is why I estimated the costs of variants of the EDM-2011 baseline inwhich natural gas was used instead of biofuel. Still the costs are far too high. Even with natural gas instead of biofuels, the system is totally uneconomic.
According to EDM-2011, the peaking capacity needed is 26 GW to meet the peak winter demand, but that is with no reserve capacity whatsoever. Of course that would be unacceptable. The system would need about 33 GW of gas generating capacity to meet peak winter demand with 20% reserve capacity.
What do you mean by “many”? Where are they? What would be the generating capacity? What would be the total cost? Without these sorts of essential details I regard comments like these as simply RE advocates’ “twaddle”. By the way, $60/kW is a complete joke. If that was true, it would have been done long ago. I was involved (peripherally) with some of the Pacific Hydro work in the 1990’s. Very few of the sites are economic.
Neil, you frequently make unsubstantiated statements like these. But, where is your cost estimate? How do you excavate below the existing turbines to install the pumps. Does Pacific Hydro shut Tumut 3 down for say three years? Who pays for the loss of revenue? Calculate the Net Present Value of three years loss of revenue partially offset by increased revenue for the remaining life of the plant – if and only if the plant can buy power for pumping at about ¼ the price it can earn from selling peak power. That would mean it would have to buy power at night when the Sun has gone to bed! Of course, this is totally impracticable if renewable energy is supplying the power. I’ve explained why many times before on the Pumped hydro thread and also explained in the critique of EDM-2011. I don’t understand why you keep repeating the same nonsense, despite having been corrected time and time again.
By the way, if the pumping capacity of Tumut 3 was doubled, we’d add about 400 MW of pumping capacity (of recoverable energy).
Your 500 GWh that you continually repeat and I continually correct you on is wrong!!. That is WRONG!! Repeating this nonsense over and over again, even though I’ve corrected you over and over again seems to be intentionally misleading other readers. You know better, but continue to, apparently, attempt to mislead the readers. Refer to numerous comments on the Tanatangara-Blowering Pumped Hydro thread, e.g.:
http://bravenewclimate.com/2010/04/05/pumped-hydro-system-cost/#comment-133008
http://bravenewclimate.com/2010/04/05/pumped-hydro-system-cost/#comment-133727
http://bravenewclimate.com/2010/04/05/pumped-hydro-system-cost/#comment-136110
http://bravenewclimate.com/2010/04/05/pumped-hydro-system-cost/#comment-149007
http://bravenewclimate.com/2010/04/05/pumped-hydro-system-cost/#comment-86108
Assume the Tantangara-Blowering pumped hydro project could have a reliable and sustainable energy storage of 40 GWh to 50 GWh .
Neil, I didn’t find anything in your comment that would suggest to me I need to change anything in the lead article for this thread.
Ender, @ 12 February 2012 at 1:55 PM
It seems you have not read, or at least not carefully, the EDM-2011 paper and my critique. Therefore, I’ll leave getting into detail with you until you have done so. Here are a couple of brief points in the meantime:
EDM-2012 assumes parabolic trough, not central tower. So you have chosen the wrong numbers.
Secondly, there is a great deal involved in converting figures fro EU and USA to Australia. That is why DRET commissioned EPRI to do that. The reference for that is:
http://www.ret.gov.au/energy/Documents/AEGTC%202010.pdf
I suggest you to study that report carefully.
Some of the figures in the EPRI (2010) report were then adjusted as a result of stakeholder group meetings conducted by ACIL-Tasman commissioned jointly by DRET and AEMO. Then Worley Parsons, also contracted by DRET and AEMO produced the report and the numbers in the spreadsheets now available on the DRET web site.
Any other nubers you come up with will have much less credibility than these. So I would not be interested in discussing whether these numbers are right or wrong. These are the numbers the government is using for its modelling, so they are the numbers I will use too.
You need to be careful as to whether the figures you quote are:
• gross (generated) or net (sent out)
• 2009-10 A$ or some other year and some other currency
• before or after tax
• include or exclude Interest During Construction and Owners costs
• what capacity factor you are using
• the discount rate
• the book life of the plant
• many other variable too.
All these make a big difference to the outputs.
The figures I’ve used are prepared on a consistent basis. You’ll understand all this a lot better after you have read the EDM-2011 paper carefully, my critique carefully, the EPRI(2010) report, and the ACIL-Tasman (2010) report.
Neil Howes,
There is another point I should make about Tantangara-Blowering Pumped hydro scheme. I don’t think it is viable, the reviewers didn’t think it would be viable, and I expect no experienced engineer really believes it would be viable. Not even the smaller version linking Tantangara and Talbingo would be viable.
The three tunnels for Tantangara-Blowering are each 53 km long and 12.7 m diameter. The weight of water, as I explained on the Pumped hydro thread in the first link provided in my earlier comment to you, is about 20 million tonnes in each tunnel. This flows at 3 m/s. Consider the inertia and the forces that would be required by the pumps to start and stop 20 million tonnes moving at 3 m/s.
For comparison, Wivenhoe is 420 m tunnel length and Tumut 3 is 488 m length of steel pipes. The weight of water is 24,000 tonnes in the Wivenhoe tunnel and 12,000 tonnes in each Tumut 3 pipe. Therefore, the weight of water to be stopped and accelerated each time the pumps stop and start is ~1000 times more for the Tantangara-Blowering idea than for the Wivenhoe and Tumut 3 plants.
Peter Lang, on 12 February 2012 at 4:43 PM said:
I was referring to the total long term storage available based on the active storage volume of Tantangara( approx 250,000ML) generating 9GWh/h at a flow rate of 4000ML/h(60h x 9GW=540GWh).
You were referring to a pumped hydro scheme operating 6-8h/ night with base-load off-peak power.
With a large amount of renewable wind power excess wind energy can occur for several days or weeks, and low wind periods occur for several days to a week(ie up to 90h max pumping).
The economics of running pumped hydro are determined by the total number of GWh stored per year and the difference in cost of power used for pumping and value of power used to avoid biogass consumption. Any potential “spilled” or curtailed wind energy would be available to be used by variable output pumping turbines at very low cost. This would occur during high wind periods and moderate wind periods with high solar output(about 2500h/year). The EDM-2011 base scenario has a high biogass demand concentrated in the winter months which would soon exhaust ever 500GWh of pumped storage. Using less CSP capacity located mainly in north QLD and more wind capacity would allow more opportunities to recharge pumped hydro in the winter months.
Uprating Australia’s hydro capacity using existing dams, especially in TAS has not been required because of the limitation of the Bass-link. It should be considered as an option rather than overbuilding expensive CSP.
IMO it is no longer politically possible to build a large hydro dam in Tasmania. The 180 MW Gordon-below-Franklin got knocked a quarter century ago and the main protagonists (Bobs Hawke, Brown et al) are still around. A couple of new irrigation dams have 2 MW generators.
Interestingly the 442 MW Strathgordon dam has vacant mounting slots for two 150 MW turbines and generators. That dam really only fills in La Nina periods like now and is chronically low in other years. That means pumping could be impossible at times of strong river flow.
The normal 500 MW export limit on Basslink is something they should have thought of earlier. I can see the day coming when even Tassie will consider a small NPP to keep the smelters going in dry times. That could be soon as Bass gas is fading fast.
Peter Lang – “EDM-2012 assumes parabolic trough, not central tower. So you have chosen the wrong numbers.”
Actually I did no such thing. I said that this number was for a power tower that was likely to be more expensive that mature troughs.
Perhaps you now should have a better look at my post.
Ender, I saw your post. Sorry for not correcting on every single wronng thought that enters your head. Frankly, I can’t be bothered since you can’t be bothered reading the background. If you’d read any of it, you’d know that once again you are dead wrong and wouldn’t have made such a silly comment. Look it up for yourself.
There is a long history of your total confidence in your beliefs – mostly wrong, like this one.
JN, The Tulley Millstream hydro project at Tulley Queenslad was also killed off by Green opposition in about 1990. I worked on that project.
Peter Lang – “Frankly, I can’t be bothered since you can’t be bothered reading the background. If you’d read any of it, you’d know that once again you are dead wrong and wouldn’t have made such a silly comment. Look it up for yourself.”
I posted a reasoned post showing where you inflated figures by a factor of two going from CSP with 7.5 hours storage to 15 hours storage.
Andasol-1 is a trough plant with 7.5 hours storage. I am sorry the reference was wrong. It was completed in 2009.
ref 3 should be http://en.wikipedia.org/wiki/Andasol_Solar_Power_Station
I presented a far more likely cost growth to 15 hours storage. I also agree with Neil that costing this study is premature. Nobody would build massive CSP plants in remote areas when the local solar insolation in most capital cities or very close and still within the present electricity grid is good enough.
You obviously also missed me saying that I consider that a few nukes could be far more cost effective.
MODERATOR
I have let this run for a while but the exchange is becoming inflammatory. Please desist. PL has been likewise advised.
Graeme Parker – “However the bioenergy proposal requires dedicated infrastructure for feedstock supply, transport, conversion and storage over a full year, but will only be utilised for short periods during winter. See page 5 and 13 of the pdf.”
I saw that however do you really think that it would inflate the price by 5 times? The amount of time a resource is used does not affect the capital cost.
@ Ender
For estimates of biomass capital cost, refer to the reference of page 24 of the critique:
http://www.iea.org/techno/essentials3.pdf
I’m not sure how you would precisely estimate the Elliston et al bioenergy proposal without more detailed project specifics, but the $5,000/kW appears a reasonable first estimate given the novelty of their proposal. Can you provide a better figure, assuming the use of bioenergy-fired turbines with storage and transmission, or point to a project that approximates the Elliston proposal?
Ender, @ 12 February 2012 at 11:24 PM
No, you didn’t show I’d inflated figures by a factor of two. You were wrong! (I explained @ 12 February 2012 at 5:13 PM why you cant simply compare US or EU costs with what the costs would be in Australia, without ensuring all costs are on a comparable basis and you have used appropriate factors for converting from US or EU costs – e.g. labour rates and labour productivity- to Australian costs}.
You were also wrong when you said, and then repeated in a subsequent comment, paraboloic trough is cheaper than central tower.
Since you didn’t admit you were wrong and provide the figures for other readers, I shall. The capital cost unit rate in in 2009-10 A$/kW “sent out”, provided by EPRI (2010) for “sent out”, ACIL-Tasman (2010) converted to “sent out”, and DRET (2010) average of high and low cost converted to “sent out” are:
CST Parabolic Trough (no storage) = $5,677
CST Central Receiver (no storage) = $4,559
CST Parabolic Trough (6 h storage) = $8,751
CST Central Receiver (6 h storage) = $6475
These costs exclude Owners costs and Interst During Construction.
You can also see how the conversion to 15 h storage was done at the bottom of the Excel spreadsheet -which, by the way, you said in your earlier comment you had “looked at”.
I look forward to your apology for wrongly stating:
– Parabolic Trough is cheaper than Central Tower, and
– I had inflated the costs by a factor of two.
MODERATOR
Inflammatory comments have been edited out. Please calm down. Ender has also been advised to desist.
Graham Palmer,
Thank you for your response to Ender’s comment about the capital cost of a biofuel energy system in Australia that could deliver the biomass reliably through all seasons and decade long droughts, store it or the fuel for use of just a few short periods in winter, distribute it at the rate needed to generate 24 GW of power for a few days at a time (outside the hours when the sun is shining strongly).
I’d also refer readers to the Grattan Institute report which quotes figures of $3,500/kW to $5,500/kW. But that is for plants running all year at about 70% capacity factor. That is not what the EDM-2011 study envisages. Their study invisages 13% capacity factor with use just in winter. This requires enormous storage and distribution capability. The storage requirement is equivalent 290 PJ if it is to be stored as biogas. This is more gas than the NEM uses per year to generate electricity and it is all required to be delivered over a few short periods in winter. Surely, Ender must be able to recognise that this will cost much more that a plant running at 70% capacity factor.
The Grattan Institute report explains that the $3,500/kW figure is a projection for large biomass plants but the haven’t been shown to be possible in Australia because the logistics of the supply chain are not shown to be feasible. The $5,500/kW figure applies for plants of a size that may be feasible (at 70% capacity factor), but I suspect even this figure would be an underestimate – except for a few ideally placed plants.
See Figure 8.6 and accompanying text in Grattan Institute (2012):
http://www.grattan.edu.au/publications/125_energy__no_easy_choices_detail.pdf
Ender, in response to your post yesterday @ 1.55 PM re the high CSP costs, we debated this for some time and it is important to explain how the data were derived. As Peter points out, all the costs are based on EPRI data prepared for the Australian government in 2010. These were chosen because the EPRI calculations use international benchmarks but adjust them to reflect the local environment. This makes them the best estimate for assessing costs in this country. It means that direct comparisons with plant costs elsewhere may be reasonable but irrelevant given the decisions to select EPRI data as the benchmark.
EPRI did not assess a cost for a CSP plant with 15 hours storage (as advocated in the document being critiqued) so it was necessary to take the EPRI cost without storage and the cost with 6 hours storage and estimate the increase to 15 hours. As Peter has pointed out, this calculation was included at the bottom of his spreadsheet. In simple terms, the cost of the base plant (1x solar field plus power plant with no storage) was taken from the cost of the plant with 6 hours storage which including the same power plant but additional solar field to supply the storage heat. This difference gives the additional cost for each hour of storage by dividing the difference by 6. Multiply this additional cost per hour by 15 and adding back the base plant gives an estimate for the cost of a plant with 15 hours.
If you believe any of this logic is flawed then please let us know.
I’ve been quietly following this article and subsequent comments, and I just thought I’d say well done Peter Lang on a stellar piece of work. I think it’s disappointing no one who has commented on it in the negative has actually used the excel sheet provided to test their assumptions about costs, and how this would affect overall cost. One can bicker about the details, but in the end unless that has significant implications for the total cost, the overall conclusions we can draw from this analysis stand.
Also, I provided a link to this critique on this newmatilda article on the Grattan Institute report, in response to a comment by Mark Diesendorf.
Actually, apologies to Ender, who did use the Excel sheet to test assumptions. I note that $334 billion is still > 4 times the current cost of electricity, and this is using some very optimistic assumptions.
Martin Nicholson, Thank you for providing that clear explanation. And credi to you for the elegant method.
Tom Keen, thank you for the comment and thank your for the well explained post on Matilda. I think your comment here cuts to the chase of where I feel the effort should be placed to find any holes in the methodology, assumptions and calculations in the critique of EDM-2011.
… and suggest (and defend) a more appropriate methodology, if you think you have devised one.
Just back from NZ Peter but congratulations on producing a great piece. You must send a copy to EDM.You may remember, I noted how many European and other countries were reducing or removing subsidies for the renewables during my Ockham’s Razor talk on Sept15th last year. Other countries have obviously concluded that the renewables are too costly. Your piece has certainly nailed that for us in Australia. And former chairman of ABC, Maurice Newman [I met him here in Port Lincoln late last year and gave him some reading material on nuclear etc] was recently quoted in the Australian talking about wind farms. He noted, “They fail on all counts. They are grossly inefficient, extremely expensive, socially inequitable, a danger to human health, environmentally harmful, divisive for communities, a blot on the landscape and don’t even achieve the purpose for which they were designed,namely the reliable generation of electricity and the reduction of CO2 emissions.” It’s a pity he couldn’t have said it while chairman of the ABC. I’ll be using part of it in my talk to the Retired Engineers Group in Adelaide on 7th March. Is it OK with you if I make reference to your study as well? Cheers. Terry
Taking a deep breath ……
Peter Lang
The figures you are using are “RENEWABLE PLANT CAPITAL, O&M, AND COST OF ELECTRICITY SUMMARY (Near Term, 2015)”
In fact they are projections of what they think CSP will cost in 2015 which could be completely wrong. Right now troughs are a mature bankable technology that are much cheaper than central receivers that are just getting off the ground. You only have to look at the 20MW Gemasolar (Ref-1) plant at nearly 500 million compared to the Torresol-1 that came in at 380 million for 50 MW.
If EPRI thinks that in 2015 central receivers will be cheaper than troughs then they may be right however they could also be dead wrong. I do not agree that these figures are the last word and are any more accurate than any other.
In the context of the references you were using I was dead wrong and I apologise however in the real world of 2010 which this study is based on I am right. Central Towers at the moment are far more expensive.
To your second point the inflation of prices. If we use your 2015 figures of $8751 for 6 hours storage then your figure of approx $13 000 is not double this. I cannot get your spreadsheet at the moment to detail your cost inflation however you have not commented on the real world figures of Torresol-1. You cannot simply brush it off as not applicable. Neither EPRI or DRET have actually built a solar thermal plant and are guessing at what they think it will cost in 2015.
Conversion factors or local factors would not inflate the real USD$380 million cost to AUD$650 million dollars that your figure would have the 50MW plant costing. My pretty generous figure of $500 million is far more justified as Australia has extensive experience with solar thermal and we have in fact invented and are the patent holders of some of the key technologies involved. I think subsequent plants would quickly drop in price from this as we would have to build a lot, justifying local industries and jobs.
To justify your higher figure you would also have to prove that doubling the storage requires doubling the receiver field. You would also have to prove that if doubling the receiver field was required then this would double the cost. If the authors has been aware of the DRET study I am sure they would have used Central Towers as then when you went to prematurely cost them you would have had to use the figure of $6475 to start your cost inflation. BTW your figure for CSP is double this figure.
You have also not answered properly that this study is not really one for costing.
http://theenergycollective.com/nathan-wilson/58791/20mw-gemasolar-plant-elegant-pricey
Martin – “These were chosen because the EPRI calculations use international benchmarks but adjust them to reflect the local environment. This makes them the best estimate for assessing costs in this country. It means that direct comparisons with plant costs elsewhere may be reasonable but irrelevant given the decisions to select EPRI data as the benchmark.”
I am sorry however I do not accept this. If the had EPRI data of averages of real world builds and there is not shortage of trough plants to choose from then it would be a bit more believable. As it is it is a guess by EPRI of what they think CSP will cost in 2015.
“In simple terms, the cost of the base plant (1x solar field plus power plant with no storage) was taken from the cost of the plant with 6 hours storage which including the same power plant but additional solar field to supply the storage heat.”
However you are making a large assumption that the increase in storage cost in linear. You don’t know for instance that the receiver field included in the EPRI cost can actually charge a 15 hour storage in Australian conditions. There may not have to be a linear increase in receiver size with the increase in storage. Also you do not know, or have not supplied any references to prove, if an increase is required then this will linearly increase the cost. I would have thought a reality check with a trough with 15 hours storage would be a good idea. You supplied none of this.
You would have actually been better, and on firmer academic ground, to use the firm ‘known” figure for 6 hours storage and supply a caveat at the end to the effect of the difference in storage rather than arbitrarily inflating the price given that you do not know enough to do this.
Barry Brook – ” and suggest (and defend) a more appropriate methodology, if you think you have devised one.”
I did suggest one of breaking down the cost in an accepted way and then increasing the components.
I have also suggested another of using the figure from the references Peter had with caveats. I think $8741/kW for a trough plant with 15 hours storage will not be too far from the money when one is built. However I do not think that this will happen any time soon. 7.5 hours for a trough plant seems to be the ‘sweet spot’ economically.
Central Receiver plants like Gemasolar, that use much higher temperatures, will probably go to 15 hours storage as the tanks will be only a bit larger than the trough plant’s 7.5 hour tanks. This may be the ‘sweet spot’ for central receivers with molten salt working fluid.
This is all the more reason why any costs projected from this study are unlikely to be valid hence my objections. This is echoing my objections of a year ago when Peter leapt upon very very preliminary results from the AUSWEA wind study. He is sometimes a premature coster 🙂
One of the interesting things about these sort of plans is trying to get a handle on the scale involved. I quickly ran the numbers on the storage required to store 290 PJ of biofuels;
1) Assume biofuel is a liquid at 30 MJ/litre, equates to 9700 ML, or 70 times the total storage capacity at Shell’s major distribution facility in Melbourne (Newport, 22 hectare site with 40 tanks).
or 2) Assume biofuel is CNG at 200 bar at 10 MJ/litre, equates to 29,000 ML, or 900 pressure vessels, each 100 metres high, 20 metres diameter.
http://www.shell.com.au/home/content/aus/aboutshell/who_we_are/shell_au/operations/downstream/supply_distribution/newport/
The really interesting part of this study shows that in my opinion nuclear could play a part. As I said in my first post the optimum low cost solution might be nuclear baseload with renewables.
For instance if we called Korea today and wrote the same contracts that the UAE got for 60 billion we could have 6 nukes in 8 -15 years. They could sit at 90% CF in NSW, Victoria and Qld delivering cheap baseload and underpinning the whole grid.
A call to Torresol and the backers of Andasol-1 for some of their CSP plant with 7 hours storage, off the shelf, would see these deployed throughout Australia for about 350 million a unit. They do all the load following and some of the peak with storage.
The storage can also be filled from curtailed wind or nuclear simply by adding an efficient inductive heater to heat the salt and put it back in the hot tank. In this way the CSP plants could have oversize salt tanks that are partly filled by other sources. In short the molten salts could partly replace pumped hydro as reserve.
We can re-power our wind farms and build some in Queensland with some transmission lines. Existing wind farms can have 10MW monsters built right over them.
Most of the bio-fuelled gas turbines would go away along with the unrealistic load on our biofuel resources. OCGT would be only used for peaking as they are now.
With efficiency gains thrown in this is a plan for a really resilient grid at quite low cost. I don’t think that we could have a 100% grid and my first impression of the paper was the 23GW of gas turbines. If we need that then really we should not be doing it. It also unfortunately. and in peer reviewed accuracy, sort of confirms 100% backup of wind which is annoying as I have been saying the exact opposite for many years.
(Deleted inflammatory remark)
How about you get the model, create a NPP and model and cost a more realistic solution including nuclear. Could be a peer reviewed paper in it somewhere.
Ender — While an electric heater is certainly efficient, do recall that a thermal store is used (in this application) to energize a Rakine cycle generator. So about 2/3rds is lost to heat.
Far more efficient, it seems to me, is to attach a thermal store to an NPP. On some othr thread I did an actual (but first approximation) LCOE analysis. The conclusion was that a mostly NPP grid could economically support a modest addition of Solar PV, perhaps as much as 30% of nameplate.
If cost effectiveness is a goal along with a reliable, on-demand, low carbon electricity supply I see no room for wind turbines nor solar thermal projects.
Ender, @ 13 February 2012 at 12:34 PM
The DRET cost projections for 2015 are as good as we have. EPRI uses all the best sources of information and projections available (EIA, IEA, NREL, DOE, latest contracted prices, latest projections, etc). These are the figures the government is using in its modelling and they are the basis of the recently released Energy White Paper. Therefore, I’d take their estimates over yours. Of course projections can be wrong. Renewable energy advocate’s estimates (actually just wishful thinking) have been underestimates for 20 years. Would you seriously suggest I take your figures rather than the Australian government’s quoted figures? Imagine if I’d used figures I’d preferred.
Wrong again. There is no inflation component in the figures. All cost are constant 2009-10 A$. I.e. “real”. I suggest you read and understand the paper (and the EPRI (2010) report, and the ACIL-Tasman (2010) report, and the DRET (2010) figures and notes.
If you are talking about the cost multiplier from 6 h storage to 15 h storage, the cost multiplier is 1.53 based on the DRET figures (and using Martin Nicholson’s elegant method) or 1.75 based on the NREL SAM figures for CST Parabolic Trough https://sam.nrel.gov/cost. But the NREL figures are much older and not necessarily appropriate for Australia. So, clearly, the DRET figures are the ones to use.
Ender,
Can you have a word in the ears of Mark Diesendorf and the BZE chaps?
Now, I’d like to ask you to state your gut feeling as to what the mix would be to give us least cost, low CO2 emission electricity generation in the NEM?
My feeling is, for the 2010 demand, 73% nuclear, 15% natural gas, 6% hydro, 0% pumped hydro, 5% wind, 1% PV and 0% CST. That would give CO2 emissions of 10% of the NEM’s current emissions intensity.
If we want to reduce the emissions intensity further, I am not sure what would be cheaper to replace natural gas: a little more nuclear and pumped hydro, or CST.
What does your gut feel tell you. You can play with the Excel file linked at the top of the thread to test your ideas. You can get the inputs for nuclear from EPRI (2010) or ACIL-Tasman (2010) – remember to convert to ‘sent out’. Use 8% auxiliary load to make the conversion.
http://www.ret.gov.au/energy/Documents/AEGTC%202010.pdf
Ender, @ 13 February 2012 at 3:05 PM
How about you do that yourself. You’d learn a great deal by having a go at it yourself. Remember the saying:
You learn from the experience, not from reading what others have done.
Graham Palmer,
I wonder what those pressure vessels would cost? How thick would the steel have to be?
DBB,
It would be interesting to compare the cost electricity for zero emisisons options to provide the difference between baseload and peak. Some options are:
wind and CST with thermal storage
nuclear + pumped hydro
load following nuclear
nuclear + some form of direct storage (eg heat, hydrogen or something else)
My gut feeling is nuclear plus some load following nuclear up to total capacity around 20% above average demand, plus pumped hydro and perhaps some CST with thermal storage.
Peter, put an order in the fax for a thousand of these 🙂
Graham,
Thanks. I guess we also need to upgrade the highways 🙂
What would that cost? $4 million per km?
Or electric trains? What is the cost?
Train drivers? Whats the cost. Up in the Pilbara they are now charging Rio Tinto $250,000 per annum and they have to do nothing. That’s $250,000 pa to sleep. Add that cost to the cost of biofuels.
Oh, by the way. How would we transport these pressure vessels to the sites where they are needed? Would we have to pull down and re errect all the overhead bridges along all our highways?
What’s the cost of that?
Peter, might be easier to stick with bio-ethanol 🙂
Peter Lang – “The DRET cost projections for 2015 are as good as we have”
What projections are better than reality? You have the costs for a trough built in 2009 with 7 hours storage of 380 million. Do you think that it is reasonable that a similar plant with 15 hours storage would cost 650 million, almost double, to build in Australia? This is a good reality check that must be applied to projections.
Just because the figures are used by Australian government does not make them true. Governments have a long history of rubbery figures and bad projections. Just to re-open old wounds you only have to look at ABARE’s oil price predictions.
http://www.aph.gov.au/senate/committee/rrat_ctte/completed_inquiries/2004-07/oil_supply/submissions/sub135b.pdf
“If you are talking about the cost multiplier from 6 h storage to 15 h storage, the cost multiplier is 1.53 based on the DRET figures (and using Martin Nicholson’s elegant method) or 1.75 based on the NREL”
However you are mulitplying a guess by a figure plucked from the air, doubling the uncertainty. Exactly what are the error bars for the EPRI figures? I would guess 20% either way at least. What is the error bar for your guess at a multiplier? You have not designed, built or operated at CSP plant as far as I know. Who did you or Martin consult in the industry about the design of CSP plants and whether increased storage linearly increases the cost.
Please post the references you used including real world costs of CSP plants with 7 hour and 15 hour storage.
Finally as you are posting this analysis as a reference and no doubt going to be using it to bag renewables it is not valid to be using for a basis for costing. The CSP plants are concentrated in distant areas and not in a real world configuration. The wind is highly correlated as it included little north-south separation.
Your analysis is a classic case of GIGO. As to your other questions. Yes I will try to obtain the code and maybe model some more real world scenerios.
My gut feel for a mix is 20% nuclear, 40% CSP with 7 hours storage, 20% wind and 20% peaking with gas turbines. This way you can use off the shelf nukes rather that trying to get load followers as your mix would need. It will take long enough to build the conventional nukes let alone modifying them. In the 10 -15 years the nukes are being built the crash CSP program would be almost complete as it takes only about 3 – 4 years to build a CSP plant.
Ender,
You are not taking any notice of what I’ve said in previous comments. Please reread my comments then read the EPRI (2010) report and the AECL (2010) report. It is mind-bogglingly frustrating that you have almost no understanding of what is ivolved with making numbers comparable. When you have got some background, then it might be worth discussing.
Ender,
Your baseless assumptions continue. First, load following nukes have been operating for decades.
Second, you don’t need load following nukes to supply 73% of the 2010 demand.
Check your facts before making your utterances.
Ender, nuclear is far cheaper than the figures you are quoting for solar. Nuclear is only $1,500/kW. So why not just go with nuclear?
Peter Lang – ” It is mind-bogglingle frustrating that you have almost no understanding of what is ivolved with making numbers comparable. When you have got some background, then it might be worth discussing.”
From ACIL-Tasman page 25 – your reference.
“In regard to the uncertainty around the capital cost estimates provided, we note that EPRI suggest the upper and lower limit of +/- 30%. We assume these to be the extreme bounds, which suggest that +/- 10% will cover a reasonable range of the uncertainty. In fact if we can assume a normal distribution with extreme of +/- 30%, then a range of +/- 10% roughly covers one standard deviation on either side of the central estimate – this does not seem unreasonable.”
EPRI have no qualms about admitting the uncertainty in their projections. ACIL-Tasman seemed to have rationalised them to 10%. Is either of these figures in your paper? And please note they are capital cost ESTIMATES – with 30% uncertainty. These are really the best figures you could get?
Now to your fudge factor – what is the uncertainly in that? I am yet to see that answer.
Additionally if you think that the 2015 figure is so certain and the best available why did you not use the 2030 range just a bit below it? It is a lot lower – hmmmmmm wonder why it was not used.
(The comments to which you refer have been edited out.
Secondly I have not, am not and never have said that nukes cannot be load following. It is just that they need modifications to run in this mode. The cheap Korean AP-1400s do not have this modification. Also the deal with UAE is 60 years. Load following nukes have a shorter lifetime than baseload nukes. If you want nukes fast get the off the shelf ones and let the generators that do load following better (CSP) do it.
Also please explain this:
http://www.world-nuclear.org/info/inf40.html
” The high reliance on nuclear power in France thus poses some technical challenges, since the reactors collectively need to be used in load-following mode. (Since electricity cannot be stored, generation output must exactly equal to consumption at all times. Any change in demand or generation of electricity at a given point on the transmission network has an instant impact on the entire system.”
France has some 75% of its generating capacity as nuclear. This is very close to your 71%. How is it possible the you can claim that your 71% nuclear does not need load following nukes.
If they are not needed why do the French have them – is it just a French requirement?
MODERATOR
I am not on-line 24/7 and so sometimes there is a delay in the moderation. The inflammatory remarks on bothe sides have now been deleted. A warning was given to both you and PL yesterday.
Ender,
France has some 75% of its generating capacity as nuclear. This is very close to your 71%. How is it possible the you can claim that your 71% nuclear does not need load following nukes.
Actually, only 50% of Frances ‘generating capacity’ is nuclear.
http://www.world-nuclear.org/info/inf40.html
If I take France’s total consumption and divide it by their total capacity they have about a 47% utilization rate.
The average utilization rate in Australia varies from 52-56%.
http://www.ret.gov.au/energy/Documents/facts-stats-pubs/Energy-in-Australia-2011.pdf
If I do the same math on US generation average capacity factor is 43%
http://ww,eai.gov/totalenergy/data/monthly/pdf/sec7_5.pdf
I would hypothesize that Australia’s over-sized Aluminum industry makes it a much more ‘base-load’ friendly country then either France or the US.
Ender,
Of course I recognise there are uncertainties with any estimate and any projection. I expect the uncertainty on the EPRI figures are probably much higher than +/-30%. To illustrate, two cost estimates for the Innamincka to Sydney transmission line, done two years apart, had stated a similar uncertainties (about +/-30% I think from memory). But the second estimate was more than double the first. That was because the first was based on very old US data. Just because there are uncertainties does not mean we should use some bodgy number an RE advocate would prefer we use rather than the central figure for the most authoritative estimates available. I realise there are uncertainties. I also realise the uncertainties are highest for biofuel, then CST, PV, wind, nuclear, gas, coal in about that order.
I think you just like to argue about irrelevancies. You wont do any cost estimates for yourself, so you don’t understand. You pick out silly points to argue about, but cannot get your head around the whole issues from a top down perspective.
Until you have done a proper conversion for the figures you want to use, to make the properly comparable with the EPRI / ACIL-Tasman / DRET figures for Australia, and you have provided your methodology so we can review it, I am not interested in taking you seriously.
BTW, yes, with nuclear supplying 73% of electricty (that is 73% of MWh not 73% of MW), nuclear would need about 3 GW of load carrying capacity. By the time we get to 20 GW of nuclear, 3 GW of load carrying nuclear will be nothing. The French have been doing it for decades. I wonder if you can get your head around the big picture here or you will find some other minor blemish in how I’ve explained this to nit pick about).
The above statement is based on the following assumptions:
peak demand in 2010 = 34 GW (approx)
minimum demand = 18 GW (approx)
Nuclear capacity = 20 GW
Nuclear capacity factor = 85%
Average power = 17 GW
Power output when all units are runiig at full power = 20 GW
Load following capacity needed to generate only 17 GW = 3 GW
Easy, as France demonstrates every day:
http://www.rte-france.com/fr/developpement-durable/maitriser-sa-consommation-electrique/eco2mix-consommation-production-et-contenu-co2-de-l-electricite-francaise
Luckily, the French have almost no wind and solar power which would definitely complicate matters and raise the cost of electricity if the generators had to cope with their unreliable and fittish generation.
I do recognise that, no matter how frustrating, your comments do stimulate discussion that provide a lot of information that probably help others to understand more about what is behind the assumptions and analyses.
Ender,
Almost every statement you make starts from a wrong assumption or wrong premise. You clearly have not read my paper of the main references I’ve provided carefully, with an intention to understand them.
So what, the LCOE calculations for fossil fuel and nuclear assume 40 year life, for solar CST and PV 30 year life and wqinf 25 year life.
We know nuclear and fossil fules last longer than the assumed life. It is almost cerain the solar and wind will not be economically viable for anywhere near their assumed life. So there is a majoir uncertainty for you on your pet RE’s. how have you handled the uncertainty on that? Perhaps you should dounb;e the estimates for CST on that basis. And double the capital and operating cost of CST for their remote loacations. Where is the uncertainty in your estimates? Hmmmmmm?
WRONG!. Again!. France generates about 75% of its electricity from nuclear. Nuclear is not 75% of its electricity generation capacity. You are confusing MW (power) and MWh (energy). A basic misunderstanding.
Silly question. Not worth answering.
If you would bother to read the paper and understand it before making your baseless comments and if you would bother to do any number crunching yourself, I would not be so dismissive of the substance of your comments.
MODERATOR
The inflammatory statements from your comments and Ender’s have been deleted. Yesterday I asked you both to stop the pettiness. Please do so now.
Harrywr2,
Thank you for that explanation and links.
Just before Ender picks me up on it, the nuclear plants have been running nearly flat out all day yesterday. Their output changed by only about 1300 MW.
In fact all the generators are running near flat out (except the renewables, of course). I’ve heard it’s a bit cold over there at the moment, so perhaps that might have something to do with it 🙂
Slide 12 in the EDM-2011 presentation http://www.ceem.unsw.edu.au/content/userDocs/Solar2011-slides.pdf
is a clear demonstration of how renewables don’t turn up to work when most needed. Some call renewables “the unreliables” for this reason. Another appropriate Australian term that could be applied is “Bludgers”. When most needed to lend a hand they don’t turn up to work.
The output from the European wind farms and solar panels at the moment is another example. Europe is gripped by a cold spell, people are dying from the cold in large numbers, and the renewable energy contribution is near to nonexistent.
“Bludgers” is an appropriate description.
Peter, at first glance I see a few critical problems. For one, the capital costs for wind are way too high at $2744/kW – here in the U.S. costs are well below $2000/kW and falling, so I would be very surprised if costs were that much higher in Australia. Our LCOE for wind is currently about $70/MWh, about half what is calculated in this report.
http://eetd.lbl.gov/ea/ems/reports/wind-energy-costs-2-2012.pdf
http://eetd.lbl.gov/ea/emp/reports/lbnl-5119e.pdf
Second, when the output of 1.5 GW of existing wind turbines was scaled up to model the output of 23.2 GW of future wind, was statistical smoothing incorporated to account for the geographic diversity one gets from additional wind installations? If not, the assessment is greatly underestimating the reliability contributions of wind energy.
I haven’t had time to look through the other assumptions, but those are two big problems that jumped out at me.
Michael Goggin,
American Wind Energy Association
Ender,
Just tidying up one of the loose ends (statments you have made upthread that I may not have put to bed, but should), see below:
@ 13 February 2012 at 8:12 PM
No, I am not. It is a simple calculation derived from the published, authoritative figures in these two references. You can see the calculation at the bottom of the spreadsheet linked at the top of this thread.
There is no point complicating this simple analysis with uncertainty ranges when you can’t even get your head around the analysis using only the central estimates.
And where would you suggest I obtain the uncertainty figures for the NREL figures for CST?
Remember, that the solar thermal plants have not published actual cost or perfomance figures. They keep them secret, presumably because they know how bad they are.
@ 13 February 2012 at 12:47 PM in your reply to Martin Nicholson:
WRONG! Martin and I are both using what NEEDS (2007) and NREL SAM have published. If you look at a the breakdown of costs in NREL SAM here: https://sam.nrel.gov/cost you will see that the solar field costs are scaled in proportion to area of solar field (m2), and the energy storage in proportion to the kWh-t of energy storage capacity.
So, according to these authoritative sources, they are linear. Therefore, Martin’s and my estimate of 1.53 based on DRET data is reasonable for this high level, top down, cost estimate.
Michael Goggin: I think John Petersen did a very nice analysis last year on whether geographic diversity actually helps on reducing wind power generation variability: http://seekingalpha.com/article/265055-avoiding-wind-power-stocks-geographic-diversity-debunked
Do you have an analysis showing this “statistical smoothing” really reduces that variability (real-life data)? There should be plenty of data available with all the commercial wind farms in place.
Dave Runyon
Dave Runyon: Pages 19-28 here are a good resource on the topic of geographic diversity in wind output. The chart on page 26 best sums up the data.
http://www.vtt.fi/inf/pdf/tiedotteet/2009/T2493.pdf
Michael Goggin,
American Wind Energy Association
Peter Lang — I already conducteed a first approximation study of how to best meet a typical load pattern with a daytime high and a nighttime low. I used US prices for new NPPs which are more like US$6400/kW for allin captial costs. [Check the estimated cost for the Vogtle Westinghouse AP1000s.]
To repeat, the best choice for reliable, on-demand, low carbon electricity at least cost is NPPs with an attached thermal store. Then add up to about 30% nameplate solar PV if you want to. I can find no way to meet requirements cost effectively with any addition of wind turbines or solar thermal. I didn’t consider pumped hydro because the availability depends upon geography.
Hullo Michael Goggin, 14 February 2012 at 9:24 AM
Thank you for your contribution. It’s good to get the AWEA’s contribution to the debate.
I recognise your comment is based on a cursory look at my post and the EDM-2011 paper. So, I’ll correct a few misunderstandings.
I think the unit price for wind at $2,744/kW (in 2009-10 A$) may be on the low side rather than the high side. The average cost of Australian wind farms under construction in April 2010 was $2,896/kW (ABARES, 2009, Listing of Major Electricity Development Projects, http://adl.brs.gov.au/data/warehouse/pe_abarebrs99014462/EG2010_Apr.xls ) By the way, the conversion rate to the US$, used for conversion for 2009-10 by ACIL-Tasman and DRET to derive their figures, was US$0.85 = A$1.00.
Furthermore, the costs for wind have been rising sharply in Australia over the past few years. ABARES reports, every 6 months, the average cost of electricity generation plants under construction. In April 2009, i.e. one year earlier than the $2,896/kW figure, the cost of wind farms under construction was $2,317/kW and in October 2009 it was $2,591. So the cost had increased by $565/kW (i.e. 24%) in one year. These costs are an average of the six and seven wind farms that were under construction in each period.
No. Wind smoothing was not applied. EDM took a simple approach, but very wise I believe, because it avoided much of the argument that would ensue if they had used wind advocates’ assumptions about the performance that could be achieved from fictitious wind farms. We know that there is high correlation of wind farm output across the area spanned by the NEM’s wind farms – a triangular area 1,200 km east-west and 800 km north-south. The wind resource away from the area spaned by the NEM wind farms is considerably less, so I think you’d have trouble making your case that the wind output could be improved much, and certainly would not be cheaper. There are occasions where there was virtually no output from all the wind farms in the NEM for a week (e.g. May 2010). Also, the recently released Grattan Institute report points out that the Australian Energy Market Operator has recently reduced its expectation for the capacity factor for new wind farms in the NEM. By using the existing wind farm locations and factoring up their output from 1.5 GW to 23.2 GW, EDM-2011 removed one of the potential sources of argument. By so doing they were able to use the actual 5 minute output data for wind generation in the NEM, instead of some hypothetical results. I’d argue their approach was a good and appropriate simplifying assumption.
DBB,
Yes. I agree. From my, admittedly crude, estimates I do not see any evidence that wind, CST or PV help at all to give least-cost, low-emission electrricity generation. From the estimating I’ve done, they just increaee the cost of the system, the complexity, and the likelihood of power disruptions – like the 9 hour total power failure caused by the long transmission lines from James Bay, Quebec (see comment up thread @ 12 February 2012 at 12:24 PM).
Peter Lang – First of all let me concede all about French nuclear power. You are probably correct and load following will not be an issue in Australia for a long time if ever. As Harrywr2 pointed out our free ride for Aluminium will see to that.
“Of course I recognise there are uncertainties with any estimate and any projection”
However you did not mention this in your article. How about you add it?
“I think you just like to argue about irrelevancies.”
I don’t. Actually I argue because things like your analysis tends to get legs and the meme “100% RE in Australia is uneconomic” will tend to reverberate around when the details are glossed over or forgotten. Exactly like the Hockey Stick and all the crap around this in climate science. Faulty analysis of MBH98 became “the hockey stick is broken therefore global warming is not true”. Nobody who quotes this know anything about the paleo-climate or tree rings or PCA analysis however the meme remains.
The fact remain that you:
1. Use un-acknowledged uncertain figures for CSP and Biofuels
2. Applied a unknown and unverified multiplier to CSP
3. Attempted to cost an unrealistic RE scenerio that was never intended to be costed as it not representative of what 100% RE would look like.
(Deleted personal opinion on other’s motives)
Finally to also clear up loose ends. Your solar thermal calculator includes this:
http://www.nrel.gov/docs/fy10osti/47605.pdf
On Page 11 – “The reference plant (highlighted in yellow) is defined as a 100MWe trough plant with 6 hours of thermal energy storage located in southwestern Arizona. The solar multiple was set to 2.0 and SAM was used to calculate the associated solar field size”
So I take it that you entered 15 hours of storage into the spreadsheet and then linked to the SAM model with climate data for Australia and obtained the required solar multiple? If you want to claim authoritative sources then you should at least do this. If you also look on page 9 you can clearly see the reference model already has a solar multiple of 2.25. It is not linear as you claim and unless you have a caveat that your fudge factor is a linear scaling and not likely to be correct you are wrong.
As it is you cannot possibly claim that the fudge factor is from authoritative sources.
(Inflammatory remark deleted)
MODERATOR
Inflammatory remarks, personal opinion of other’s motives and petty tit for tat have been deleted from your comments and Pl’s. I ask again, that you both please desist.
Thanks to Peter Lang (and other contributors) for his tireless efforts to provide an answer to all the critiques raised here, and to those people raising them. It’s made for a most illuminating discussion thread, something I particularly value out of BNC.
Michael Goggin, 14 February 2012 at 9:24 AM
I’d like to add another comment regarding this statement you made:
We also need to add to the capital cost of wind the additional cost of transmission that must be supplied. This should, rightly, be charged to wind generators. For 23.2 GW of wind widely distributes ad you advocate, the maximum power that each wind farm can generate must be able to be transmitted to the demand centres and to all demand centres throughout the NEM. We never know which one will be generating at a given time, so its full output must be able to be transmitted. Otherwise, if the transmission capacity is not provided, the average capacity factor for wind generation must be reduced.
A rough rule of thumb for planning is to add $1,000/kW of installed wind capacity and 15/MWh to the cost of electricity generated by wind to cover the cost of transmission capacity from the wind farms. With the capital cost of transmission included, the DRET figure of $2,744/kW for wind becomes $3,744/kW.
Peter Lang — We agree except it seems for solar PV. Rather to my surprise, if solar PV becomes as inexpensive as estimators seem to think then the solar PV contribution during the sunny hours can be efficiently balanced during cloudy days. Here is NREL’s analysis using solar thermal:
http://www.nrel.gov/csp/pdfs/52978.pdf
but I already know that NPPs are less costly than solar thermal, so here is my attempt, done before I knew about the NRL study:
http://bravenewclimate.com/2011/12/07/open-thread-20/#comment-147890
Ender @ 14 February 2012 at 11:52 AM, said
I’ll address these one at a time below.
You haven’t produced any more authoritative figures, so these are still the best we have. I’ve provided all the references.
It is derived from authoritative sources using publically available data from the government (DRET, 2010). The method is consistent with that used by authoritative reference NEEDS (2007) and figures available from NREL SAM. If you have a better figure please provide it and justify it – as Martin Nicholson and Barry both asked you to do upthread. You’ve ignored that request. Therefore, I take your comments an RE advocate who knows full well you are seriously losing the debate over the costs of renewable energy to provide more than a token few percent of a low-emissions, low-cost electricity generation system.
All the scenarios should be costed. Dr Diesendorf has been stating publically that the EDM simulation demonstrates that renewable electricity can provide 100% of our electricity, it is economic and cheaper than other scenarios (such as the BZE2020 plan) (http://www.ecosmagazine.com/paper/EC10024.htm , http://www.climatespectator.com.au/commentary/cheaper-path-100-renewables ). If he is going to make such statements he should have done the cost calculations beforehand. It is highly irresponsible for researchers to be misleading the public as he is doing (for 20+ years).
I’d add, that if Dr Diesendorf, Dr Mills (solar thermal parabolic trough advocate), and other renewable energy advocates had been doing proper cost estimating for the past 20 years, they may not have been misleading the governments into providing them with so much funding for all those years. In that case we may have stopped, or at least cut back, on the waste long ago.
I don’t agree it as a “factoid”. I see it as you trying to use a diversionary tactic to try to avoid confronting the obvious. The obvious is that renewables are totally uneconomic. The cost for any given emissions intensity is far higher with renewables in the system than without. That is the stark reality staring RE advocates in the face but you just can’t bring your self to admit it. If you want to try to muddy waters by trying to talk about the uncertainties, you need to recognise that the uncertainties in the costs of renewables (and their unreliability) are far higher than for nuclear. I listed the order of uncertainty upthread and also in the section of the paper called “Uncertainties in Cost Estimates”. I did no quantify them and I see no value in doing so at this level of estimate. I also suggest that since you cannot even get your head around the basics of the central estimates, it would totally confuse you to make it any more complicated. What is clear from the central estimates is this:
You have been unable to provide anything that would cause me to change that statement. If you can provide a case, and I accept it, then I will update the document.
MODERATOR
Peter – if you haven’t read my previous comments about the line you and Ender are taking please do so now. I have, again, edited out the pettiness. Next time the whole comment goes as I can only assume you are disregarding my directions. That goes for Ender too.
One other possible issue with Elliston et al’s scaling up of wind farm performance based on data from ‘the current NEM locations’. Presumably these already-built sites represent the best available locations. Doesn’t it therefore follow that, in scaling up the current capacity by a factor of fifteen, those newer turbines will have to be in less optimal locations, and their performance will be correspondingly less? (Note I’m talking about mesoscale features with microclimatic effects like hilltops, not just weather system-scale issues). Is this a significant capacity factor risk on the downside?
Thanks for your response, Peter. Responding to a few of your points:
1. On transmission costs, see this excellent analysis published here in the U.S. that put the costs at around $300/kw, far less than the $1000/kw you are assuming (taking into account the exchange rate). I’d assume costs in Australia are comparable to the U.S. since our geographies are similar. Moreover, grid enhancements provide a number of widely shared economic and reliability benefits for power consumers, which is why in most of the U.S. the costs of transmission upgrades are allocated to consumers and not to generators.
http://eetd.lbl.gov/ea/emp/reports/lbnl-1471e.pdf
2. On wind capital costs, it would seem curious that while wind turbine costs are plummeting around the globe, they are increasing in Australia. I’m not an expert on the Australian wind market, but that seems strange to me.
3. Linearly scaling up wind output to model future installed wind capacity is not an acceptable shortcut. See the tables on pages 23-26 here for data showing that adding more wind greatly smooths out the output of the overall fleet: http://www.vtt.fi/inf/pdf/tiedotteet/2009/T2493.pdf If wind is being built in new regions that have been opened up by transmission expansion, you are also likely to see capacity factors and capacity value increase as diverse, high quality wind resources are opened up.
4. Renewable output was actually quite high in much of Europe during the recent cold spell. I checked the data last week, and Spain was producing between 9,000-12,300 MW of wind energy on Feb 6, meeting 22-45% of their total electric demand. Renewable output was also high in France and Germany:http://climatecrocks.com/2012/02/14/renewables-helped-france-avoid-freezing-in-the-dark/
Michael Goggin,
American Wind Energy Association
Elsewhere you write about the importance of using the DRET – EPRI projections for technology capital and energy costs in Australia (and other numbers having “much less credibility”). This is a pretty terrific and well reasoned study. For future wind costs, they look at anticipated improvements by 2030 (operation and efficiency, larger turbines, higher hub heights, improvement in power electronics and drive systems, wind sensing equipment, etc.). They write: “The cumulative impact of these anticipated improvements is estimated to decrease the capital cost of wind turbine installations by 35% in 2030 relative to 2015 technology” (6-64).
You have not indicated a time frame for this model build-out in your cost projections, will you be using such cost reductions for learning curves, technology development, and roll-out in your estimates? Given the high amount of wind energy projected in the EDM-2011 model, a 35% reduction (even a portion thereof) would be significant reduction in future energy costs.
I understand that in the recent cold snap Germany and Austria had to reactivate coal and oil fired generators
http://www.icis.com/heren/articles/2012/02/09/9531044/germany-fires-up-reserve-electricity-generation-capacity.html
Given that world oil production is thought likely to go into steep decline before long reliance on an oil fired generator hardly seems like a solution.
Michael Goggin,
Is not the sole consumer benefit of the grid the provision of electricity? The grid is a necessary evil, not a boon. We would do without it if we could, and would avoid expanding what we must already live with if we can.
If we must make a choice between say nuclear, for which the existing grid is approximately adequate, and wind or solar which require substantial grid development, why we would not directly allocate those very large transmission costs and other depredations to the wind or solar scenario?
Michael Goggin.
1. Just to be clear, the $1000/kW is a rough rule of thumb used in the early planning stage in the USA (I understand) and here. It covers all the costs of enhancements to the grid needed to allow the grid to handle the unreliable electricity supply from intermittent renewable energy sources. I did not use this figure in my estimates, as you will see in Appendix 2 in the attached PDF document. The $300/kW was a figure NREL came up with in a study a couple of years ago, but my understanding is it is considered in the industry to be unrealistic, and influenced by renewable energy advocacy, as is much of the work produced by the NREL. (There have also been some posts on BNC this very subject by US specialists in network planning). So I would not place much credence in the $300/kW figure. As I said, I did not use the $1 000/kW figure. The figure I used is based on studies contracted by AEMO to estimate the cost of interconnectors from Adelaide to Melbourne and Sydney. My figure assumes a mix of HVDC and AC.
2.
And therein lays the misleading statement. A wind power station is not just the cost of the turbines. It comprises much more than just the turbines. The total cost of wind farms has been going up, as the authoritative costs I provided for the actual wind farms under construction in each 6 month period show.
3.
No (i.e. I agree), not if you are at a more advanced stage of estimating. But at this stage, and to provide an estimate of the cost of electricity from a renewable energy system to power Australia, it is an excellent first step. Refinements in the estimate may raise or lower the costs, but certainly will not lower it by the amount required to make renewable energy anywhere near a viable option. Being able to understand scale is an important competency. You may also want to look at the EPRI (2010) report referenced above to see the costs EPRI estimated for wind farms of different sizes, and different average wind speeds.
That is an unquantified, unsubstantiated statement. . I want to know what is the total cost? What is the total system cost? Without such quantification, this is just more of what David Mackay calls “emissions of twaddle”.
Furthermore, I doubt your statement is true for extension of wind farms to more inland parts of Australia. See the Gattan Institute report for a good summary of this: http://www.grattan.edu.au/publications/125_energy__no_easy_choices_detail.pdf
4.
We are in Australia. The best sites for wind farms have already been developed. The 1,200 km by 800 km area in which the wind farms are located is the best resource. Any more wind farms in that area will suffer from the same wind speed correlation that the existing wind farms suffer – e.g. up to a week of near zero output at times. Outside this area, the wind resource is not as good – unless you are going to build a transmission interconnector to Western Australia. That would be prohibitively expensive.
Michael Goggin, can I urge you to read the Elliston et al. paper or the slide presentation (this provides a succinct summary), then read the PDF version of my paper (linked above). Until you have done that I think you may not be understanding the scope, wind’s role in it, and the relatively low sensitivity of the total system cost to the costs contributed by the wind component. There is little point arguing about fine details of the wind component when the largest uncertainties by far are in biofuels, CST and transmission costs.
And, please, can we focus on the costs if we are going top make suggestions about how to improve this analysis. I’ve provided an Excel spreadsheet you can down load and change inputs to see the sensitivity of your suggestion before point them. Can I urge you to try that so you can quantify the impact of your proposals on the capital cost, cost of electricity and CO2 abatement cost of the system.
Unless your suggestions will significantly change the conclusions, they are really not worth a lot of effort. The main conclusion to take fro this paper is:
Mark Duffett, @14 February 2012 at 10:52 PM
Yes. I agree. Michael Goggin, plese see Mark Duffett’s comment. I suggest the effect he has aluded to offestes the effects your are suggesting. It is intersting to note that EAMO has recently significantly reduced the expected capacity factor for future wind farms. I seem to recall (may have this wrong) Gattan Institute report http://www.grattan.edu.au/publications/125_energy__no_easy_choices_detail.pdf says something about that.
EL,
I get the impression you just want to argue for the sake of arguing. You still haven’t provided any numbers to back up your earlier statements.
What is the point you are trying to make in the quoted bit. Yes, I used the EPRI/ACIL-Tasman/DRET figures for the estimates. They are a set of figurs that have been produced on a set of consistent inputs across the technologies, they are applicable for Australia, recent, constant 2009-10 Australian dollars. And they are the costs used in the Governmen’ts recently realeased Energy White Paper and used in the government’s modelling. What more could you ask for?
Then Michael Goggin said he felt they were to high and quoted a recent US figure of $2,000/W. I said, if anything, I felt the DRET figures were more likely to be an underestiate rather than an over estimate and provided the basis for that opinion – ie the actual costs of wind farms under construction at the time the DRET figures were applicable (ie in a consistent basis). I also showed hoe that actual cost of wind farms under construction had increased 24% in the year to that date.
EL, I find you comments to be simply argument for the sake of argument. No serious attempt to provide credible costs on a basis that is properly comparable, an not real attempt attempt to relate to what is important.
I hope you will understand if I sometimes don’t respond to your comments.
I wish to clarify a comment by Michael Goggin to the effect that increasing the number of wind farms improves capacity factors. Obviously the capacity facotr of any given wind farm remains what it is. [Around here several years of data show an averagve of just under 26% despite the standard claim that the Columbia Basin area has a calcfulated capacity factor of 30%.]
Wht does happen with new wind farms further downwind is that the aggrigate energy from all the wind farms shows a smoother profile. This means the same balancing agent (hydro around here)can accomodate slightly more wind energy before the CCGTs have to kick in. I will point out that, as the legacy hydro cfannot be enhanced further, as more wind farms come in so do more CCGTs to act as balancing agents. Thus, like elsewhere, more wind promotes more natgas; or rather that is who I see the matter.
DBB your model seems to assume growing demand that has to be met by the wind-gas combination from a low wind starting point. Assume that peak demand is fixed and wind has a firm capacity of 5% for all sites taken together. Then a 20X overbuild of the peak demand will guarantee meeting that peak with curtailment most of the time.
Example; assume peak demand is 40GW and the firm wind credit is 5% then 800 GW of wind capacity will always cover it. Of course if actual demand is 20 GW and the wind is blowing hard producing say a possible 600 GW then 580 would be curtailed. At $2/w that 800 GW would cost $1.6 trillion which is the flaw in the massive overbuild solution.
JN,
I think you have a misunderstanding of what “firm capacity” means. It does not mean the wind blows all the time and you can rely on power all the time from wind farms. As we know all wind farms can be becalmed. What the firm power is is statistical measure that the planners use for planning the amount of capacity needed.
John Newlands — Peter Lang has the right of it; sometimes, indeed quite a bit of the time, the entire fleet of wind turbines with BPA as the balancing authority are completely becalmed. Indeed, this condition is often associated with both the hottest and coldest periods. Thus no amount of overbuilding can make any difference except for synoptic scale transmission [which doesn’t exist anywhere at any meaningful scale].
The so-called firm capacity allotment only makes some sense because there are also reserve requirements imposed upon the reliable (dispatchable) generators. I personally assume the worst case for wind + solar (none for extended periods) and plan enough reserve to cover that as well as the more traditional reserve requirements. Therefore I am unable to find any cost effective way to utilize wind or solar thermal in a paln for reliable, on-demand, low carbon electricity generation.
This comment demonstrates clearly what Ender (Stephen Gloor) is about.
http://www.climatespectator.com.au/commentary/house-zero-carbon-built#comment-110226
(Deleted inflammatory comment)
I posted this reply:
http://www.climatespectator.com.au/commentary/house-zero-carbon-built#comment-110226
MODERATOR
The comments on other blogs by posters (including yourself) on BNC are subject to the comments policy of that blog. BNC has a Comments Policy which is applied to posts to keep the blog relevant and civil.
Not at all, I’m very interested in your final result (and would like to encourage you to get the best possible estimate, as much as is possible given the early stages of this research). There are other ways to ask and answer a question without reference to a specific figure. I’m simply asking: 1) do you intend to follow the DRET – EPRI projection for a 35% cost reduction in wind capital costs by 2030 (page 6-64)? In effect, what is the timeline for the buildout in your model projections (you don’t appear to have one)? If you intend to depart from DRET – EPRI estimates, and assume that costs will remain flat or rise for installed wind energy over time, I assume you will want to amend the following:
Using DRET – EPRI figure of $2,744/kW (as capital costs for Australia in 2010), which you correctly reference, and assuming total build over next 18 years to 2030 (and linear cost reduction of 35% as suggested by DRET – EPRI analysis), I get a combined average of around $2263.85/kW for total buildout.
EL, if you want to do the whole analysis based on unit costs for 2030, or for half way between, or some other assumption, then I suggest you go ahead and do it. But I suggest you have to do the whole analysis on a consistent basis. You can’t just pick and choose numbers that suit you. If you do it properly and write it up you could offer it to Barry as a post for BNC.
EL, further to my previous comment, you will need to project the hourly demand profile out to 2030, and do the EDM simulation for those years, or for the year for which you select to estimate the costs. You will have to develop wind and solar power output profiles at 1 hour intervals. Then run the EDM simulation program to estimate the output from each generator.
If you want to project ahead to future years you will get into the same guess work that BZE tried.
BZE tried that approach, made what to many were unrealistic assumptions about demand reductions in future and, as a result, their analysis was largely discredited.
EDM-2011 wisely avoided that pitfall by using the actual NEM 2010 demand data. And we have the current costs for technologies, presented in 2009-10 A$ and projected to 2015. And we have those for all the technologies and prepared on a consistent basis.
I’d argue that is as good as we can do without access to the EDM model and the skills needed to drive it. But if you think there is a large error in what I’ve done, you should be able to demonstrate it, quantitatively. You haven’t done so yet, and nor has anyone else.
Of course I am making many simplifying assumptions in the crude analysis, but after 110 comments, no one has yet pointed out a significant error or provided a reasonable alternative method and substantiated it.
I urge you to have a go yourself. You make lots of suggestions about how to do the analysis better, but you haven’t thought through the implications of what you are suggesting. Until you have a go at it yourself, you will never get your head around all this.
I haven’t followed the entire debate between you and Ender, but a less expensive single tank thermocline TES system is envisioned by many parabolic trough developers, rather than the more expensive two tank approach referenced by DRET – EPRI (with 6 hours of storage). Sargent and Lundy (2003) have provided a costing for such an approach, and parabolic trough CST that incorporates many of these current and future development trends and efficiency gains.
For their base model … their technology assessment with projected cost reductions for 2020 come to a 56.2 % capacity factor, USD $3,220/kW capital cost, 12 h TES storage (and 24 hour operation). They build on an earlier National Laboratory “SunLab” study for their assessment and methodology (and reach a higher cost for their study). Solar Two is seen as a precursor to future TES development trends at the time (more here on future TES options): molten salts and ionic fluids for heat transfer (operating at 500°C or above), two tank molten salt storage system (high cost), single-tank direct molten salt thermocline system (lower cost). Sargent and Lundy also envision future cost reductions from better solar field optical efficiency, minimizing receiver and piping and storage thermal losses, EPGS efficiency, electric parasitic loads, reduction of forced and scheduled outages, O&M reductions, and more.
Take from it what you will. Sargent and Lundy envision a cost reduction of 33% between 2004 and 2020 (as a consequence of above and more). This is similar to the 30% cost reduction envisioned in DRET – EPRI analysis for solar thermal capital costs by 2030 (see page 6-56). As you have suggested, there is an asterisk near many of your projections (and uncertainties in range of 17 – 34%). With solar thermal, particularly since you aren’t looking at lower cost TES options, or cost reductions for timeline and technology development trends, it’s worth noting that this uncertainty may be larger than the amount you have indicated for the case of CST. I’m out of town next 5 days, so keep in mind when anticipating follow-ups.
EL,
You have not yet produced any costs for what you suggest is a cheaper scenario? You haven’t made any attempt to provide alternative costs on an equivalent basis to the other technologies so they can be used in a comparative analysis
As I’ve said before, suggestions are just twaddle if you support your suggestions. If you are still using the Sargent and Lundy (2003) cost projections as your source for solar costs, after it has been totally debunked (by all except the renewable advocates who still clutch to it), then your comments have little credibility.
What is the cost of the scenario you are proposing? What figures do you suggest need to be changed in the tables in Appendix 1 and 2 of the PDF? What is the justification for changing them?
The figures in the thread above clearly demonstrate that renewable energy is totally uncompetitive. You need to find a way to reduce the system cost by a factor of about seven, and make the proposed system reliable, before it could be considered a possible option.
(Inflammatory comment deleted)
You can also extrapolate from the figures in this analysis to see that renewables are probably not viable at any level of penetration. They are not justifiable
Who has debunked the Sargent and Lundy (2003) report, and on what basis? They cite a Nextant projection “that a 35% cost reduction can be achieved going from a two-tank system to a thermocline system” (p. 4-19). This is the same amount discussed in more recent peer reviewed literature: “In cost comparisons, the thermocline system is about 35% cheaper than the two-tank storage system, due to reduction of storage volume and elimination of one tank” (p. 68). You can choose to use any numbers that you want. This is your study. But to my mind, holding capital costs steady, or assuming that they will rise in the indefinite future (as you do for CSP) flies in the face of available facts, and it also flies in the face of the DRET – EPRI analysis for Australia that you recommend so highly (and which forecasts large decreases in capital costs for renewables over time due to learning curves, volume production, plant scale-up, technology development, and more). (Inflammatory remark deleted.)
EL,
You still. have not proveded a clear statement of what figures you suggest be changed in the Tables in the PDF, what they should be changed to justificatoion for whey they should be changed, nor the effect on the total system cost. Until you have a go at ti yourself, you will not understand what you are doing. Heve a go at it yourself and then the readers can discuss what you are proposing. I think waht you are suggesting is jut twaddle (as per David Mackay).
I am not persuaded, by any of the arguments presented so far, that the EPRI / ACIL-Tasman / DRET estimates for solar thermal costs are too high.
On the contrary, I suspect they are more likely to be too low rather than too high. Here are some reasons:
1. There is no allowance for the higher cost of construction and operation and maintenance which must be added because of the remote locations of the solar plants. This could conceivably add 50% to the estimated cost of electricity from these plants.
2. History shows that the projected costs of solar are continually understated. NEEDS (2007) http://www.needs-project.org/docs/results/RS1a/RS1a%20D12.2%20Final%20report%20concentrating%20solar%20thermal%20power%20plants.pdf projected that the costs of solar thermal would decrease by 30% by 2010. In fact, the opposite happened. The costs increased by 25%.
3. The expected economic life of the plants is probably greatly overstated. Ender, or was it Bilb, argued on the “Solar Power Realities” thread (or the Addendum), that the solar power output figures from Queanbeyan solar PV power station should not be used because the solar panels were too old to be relevant. They were a few years old at that stage. He said they were out of date and had been superseded by newer and improved technologies. That is, they had been superseded within just a few years. That shows how quickly these new technologies become out of date and superseded. This further indicates that the economically viable life of these technologies will be short. The same general principal applies to solar thermal, although the rate of becoming superseded and not economically viable may be a little slower.
4. Another example showing how quickly these new technologies become out of date and have to be replaced was reported this morning. Today it is reported http://www.theaustralian.com.au/business/in-depth/soon-to-expire-connection-has-earlybirds-in-slow-lane/story-e6frgaif-1226272160872 that the NBN boxes rolled out to the NBN customers in Tasmania (the first installations) have to be replaced. They’ve been superseded in just 1 year. That is likely to happen throughout the life of the NBN (but hopefully at a slower rate). The same is inevitable with all the new solar PV, solar thermal and energy storage devices being continually dreamed up.
5. These new technologies have a short economic life and then have to be replaced. So the capital cost, accumulated through the expected 30 year life of the plant, can be expected to be much higher than the initial capital cost we are currently using. Therefore, the capital cost figures we are using are likely to be too low. I suspect much too low. So the LCOE we are calculating are also likely to be too low.
6. The O&M and replacement costs will also be much higher than the renewable energy advocates are stating.
7. There is still no publically available performance and cost data for existing, commercial, solar thermal power stations (and that is despicable given the public is paying for them).
Therefore, I suspect the costs for solar thermal are more likely to be higher than lower than the DRET figures.
Given we have no more authoritative costs to use, that are properly comparable across all the technologies being considered, I can see no justification for changing the unit cost figures for either Wind or solar thermal. I can see a justification for increasing the cost of PV, by a factor of about 1.5 to 2, as explained in the post.
(The comment to which you refer has been deleted)
Again my thanks to our patient moderator. {Although the entire exchange has by now become exceeding tedious.]
The Elliston et al. claims for the potential for bioenergy are taken from Diesendorf, 2007, Greenhouse solutions with sustainable energy (book), but for those without the book, the following report contains the same information on page 80 & 81:
http://www.bioenergyaustralia.org/reports/Clean_Energy_Future_Report.pdf
There is little reason to doubt that there is substantial untapped potential for agricultural residues, and given the right market conditions, I would agree that perhaps a substantially larger market for bioenergy could be developed. Arguably Finland is the world leader in bioenergy, which uses mostly trees and forest residues for space and water heating, and increasingly for boilers in CHP (combined heat and power plants). This is similar to other leading bioenergy users in Scandanavia and Europe. As an aside, I looked at the viability of marketing wood chip space heaters a few years ago and concluded that they weren’t economic and would face enormous market challenges. However commercial appliances can be purchased in Melbourne, but few are sold and the cost of the bulk pellets is too high (pellets were $360/tonne in 2006, $475 in 2008 and $600 in 2009), which worked out at 3 to 4 times the running cost of natural gas.
http://www.unep.org/GC/GCSS-IX/Documents/FINLAND-bioenergy.pdf
The Elliston approach is quite different from the Scandavian bioenergy industry and appears to be novel (although technically feasible) and untested on a commercial scale. The Elliston energy claims are based on a simple theoretical calculation of taking the product of area x yield x energy density. Without further consideration of costs, technology, ecological impacts, economic benefits and consideration of farmers, harvesting, transport costs and logistics, processing, storage, EROI, seasonal variations, droughts, and other considerations, it is a purely theoretical exercise, and really no different to the sort of non sequitur frequently used in these exercises such as “a square 50km x 50km with solar panels could supply all of Australia’s annual energy.” What does this tell us, and what does it contribute to a serious debate about energy? Not a lot.
@Graham Palmer: Finland does get around quarter of its primary energy from forests which is roughly similar to the share of coal and gas combined. However, most of this is from byproducts of the forest industry and unlikely to be economically viable on their own (without subsidies). Also, the use of wood for CHP is rather limited to the country side. In bigger cities and especially in the Helsinki region (where I live, and which is pretty much surrounded by forests) the CHP plants run on coal and natural gas. There is some discussion of using more bioenergy in those plants, but the required amounts of biomass are so large that the area required extends much further than the usual harvesting areas in the country side.
With ‘waste’ biomass we have to ask what has been done with it up to now. I think the answer is that very little of it has been wasted. A lot of combustible material has been left to rot in situ to regenerate the soil and only the best bits taken away. That gives us timber offcuts, sawdust, vegetable oil, bagasse, chicken litter, straw, incinerator garbage and so forth. Their harvesting, million and disposal has been paid for by other objectives so they are a sideline. If they become the main objective the economics completely changes. It means using diesel and fertiliser to get a decent net energy return with EROEI > 8 if proponents of that theory are right.
On wood pellets I’ve wondered if every home with a chimney should contain a suitable stove for emergencies. If for example a frost hit Sydney the power draw for electric heating would be enormous. Every house would have several sealed bags of pellets on standby, too pricey to be used regularly. Thus the biomass would be distributed not burned in a central location.
A comprehensive study titled THE ENERGY BALANCE OF MODERN WIND TURBINES was published by the Danish wind industry in 1997. Hardly an unbiased source but the material is very detailed if rather dated.
http://apere.org/manager/docnum/doc/doc1249_971216_wind.fiche37.pdf
The result was that for onshore wind turbines the energy payback period was 3-4 months including scrapping the turbine at the end of its life. It seems unlikely that modern turbines would be less efficient than those of 1997. Assuming wind turbines last say 20 years and the paper is not serious flawed this would be an EROEI of 60:1.
EROEI is off topic for this thread. I hope you can move these last three comments to the Open Tread or the EROEI thread.
Can we please focus on trying to find errors in the lead article. To date no one, as far as I can see, has tried using the Excel spread sheet linked up thread to check the effect of their ideas on the total system costs.
I’ve had other input that suggests the area required to support the biofuel would be far higher than I’ve estimated. Perhaps six time higher. The reason being that my figures are based on UK land productivity. If someone want to look into that a start might be to look at page 8-9 in the Grattan Institute report and factor from 30 MW at 70% capacity factor to 24 GW at 13% capcity factor.
I suspect if someone wanted to think carefully about the assumptions I’ve made for estimating the transmission capacity required (see Appendix 2 in the PDF version), they could come up with a different way of looking at it. That would be a helpful contribution.
Kind of off topic, but I’m quite impressed and surprised to see Mark Diesendorf criticising the Beyond Zero Emissions “study” and acknowledging many of its blatant flaws.
Now they can’t just come back with the usual predictable retort and claim that all us scientists criticising them are all just part of the big mean nuclear conspiracy!
Luke Weston,
I agree. There was quite a heated on-line discussion between Mark Diesendorf and Matthew Wright, lead author of ZCA2020, on a number of other blog sites. In one of those he accused the BZE team (the group who produced the ZCA2020 report) of having a preconceived view of what was needed and then doing all the analysis to fit their preconceived idea.
Graham Palmer @ 16 February 2012 at 9:56 PM and
Jani-Petri Martikainen @ 16 February 2012 at 10:42 PM
Thank you for your excellent comments. Both of you cut through to the heart of the issue. And you explain it very well.
I think Graham Palmer’s comment is very relevant to the Australian situation. It seems Diesendorf and the EDM-2011 study are assuming that most of the biofuel would come from crop residues. Graham has explained the basis of the Diesendorf assumptions. This indicates that most of Australia’s crop stubble would have to be collected and converted to biofuels, stored until needed and transported to the biofuel power stations. Given that we have long droughts (the last one was 15 years), the amount of biofuel storage required might be two to five times the average annual demand.
Two other estimates have been pointed out to me. These use the figures in the Grattan Institute report. Both show that we would need to collect all the crop residue from all the grain crops in Australia to meet the EDM-2011 fuel requirements.
Graham Palmer and anyone else,
Could you offer an independent estimate of the capacity of the interstate transmission interconnectors that would be needed to achieve the EDM-2011 assumption of a [near] copper-plate transmisison system. I’ve described my approach and assumptions in Appendix 2 (in the PDF).
[…] your CO2 emissions footprintIFR FaD 10 – Metal fuel and plutoniumCarbon tax in Australia in 2011100% renewable electricity for Australia – the costPumped-hydro energy storage – cost estimates for a feasible systemAboutFukushima Nuclear Accident – […]
Do you have any efforts for solar energy?
thx
Looking a little closer at some of your assumptions, the storage costs in the DRET – EPRI assessment appear to be significantly more expensive than current estimates (and by a factor of some 500 – 1600%). Six hours of storage with a total capital cost of $2767/kW is some $461/kWh AUS (or $499.5/kWh USD). Looking at two available sources for cost estimates on storage for parabolic trough CSP plants in 6 – 15 hour range (one from EPRI), we get one source calculating these costs in $116 – 89/kWh USD range, and another in $40 – 30/kWh USD range (factoring in total direct costs for nitrate salt inventory, storage tanks, tank insulation, tank foundations, oil-to-salt heat exchanger, nitrate salt pumps, and balance of system). Do you have any explanation for why these costs are so high in the DRET – EPRI assessment for Australia?
Let’s do a “best case” sensitivity analysis using your spreadsheet for 1) updated CST costs with 15 hours storage, 2) system costs in 2030 as suggested in Diesendorf response to Lang and DRET-EPRI cost reduction factors, and 3) lower discount rate of 8.1% as suggested here (and in ATSE and University of Melbourne analysis).
Inputs:
1) CST costs: plant cost is listed as $5109/kW in your account. For updated storage cost, let’s use $82/kWh AUS (converted from $89/kWh USD) in more recent EPRI assessment (estimate for 3500 MWh storage system). For a 233MW plant with 15 hours storage (3500 MWh), total plant cost is $1.477 billion (or $6340.76/kW).
2) 2030 system costs: DRET-EPRI forsees 35% cost reduction (relative to 2015 technology) for solar PV (page 6-60), 35% reduction for wind (page 6-64), and 30% reduction for CSP (page 6-56).
3) Discount rate: 8.1% (recommended in University of Melbourne assessment to comply with ATSE analysis, 75-25 debt-equity split, 7.3% debt cost, and 17% pre-tax equity cost in Australia).
Result: $174/MWh AUS (total system LCOE in 2030, relative to 2015 costs, and using lower EPRI cost estimate for TES storage, DRET-EPRI cost reduction factors for 2030, and ATSE recommended discount rate).
Inputs:
4) OCGT: also includes $801/kW input for OCGT (from DRET – EPRI assessment page 7-14).
One recent study considers native grasses on pasture land as an alternative feedstock to food crops for biofuel production in Australia: “The non-cleared land in this extensive agricultural zone shows a promising technical production potential (266 Mt y−1) and if 15% of this land’s NPP were to be transformed into ethanol, it could replace a significant part (54%) of current Australian petrol demand” (Herr, et. al 2012).
Preliminary national resource estimates for agricultural feedstocks in Australia can be found here: “Preliminary estimates show that upper limits for second generation biofuels to replace petrol may be between 10 – 140% of our current petrol usage” (p. 12), and additional research and models are being developed with specific attention spatial distribution, climactic variability, and transportation issues (as they relate to costs), but further research is needed (based on my quick look at literature).
Grass fuel to replace half of petrol? See Cellulosic Ethanol Reality Begins to Set In by Robert Rapier. Note the quota for cellulosic ethanol was 6.5 million US gallons in 2010 but production was 0 gallons.
“Half of petrol” is a measure of resource availability. You’d convert grass feedstock into biogas for use in OCGT plants (as with agricultural residues in a 100% renewables EDM option).
EL,
I haven’t tried to follow through your calculations and assumptions. I’d make the following points:
1. I am not clear if your cost is for the storage component only or includes the solar field multiplier. If you look at the NREL SAM spreadsheet (link provided in previous comments) they provide a breakdown of representative costs. Using those the multiplier from 6 h to 15 h storage for CST parabolic trough is 1.75, i.e. higher than the 1.53 I derived (thanks to Martin Nicholson’s elegant method) from the DRET figures. From memory, this multiplier is less than we get using the NEEDS (2008) figures. All the links are provided in the lead article and my previous comments on this thread.
2. As I’ve stated before, if you want to argue to change the figures for one technology in a suite of technologies being compared, you need to make the appropriate changes to all the technologies to keep them comparable. The figures used in comparisons must be produced using the same methodology and a consistent set of inputs. I raise this because I’ve noticed you suggesting on another thread that the discount rate of 10.1% is too high and I should use 8.1%. If you want to suggest that, then you need to understand why it AEMO, DRET, ACIL-Tasman and the entire stakeholder group involved in updating the EPRI figures for use in the 2011 Energy White Paper and the Treasury’s modelling, decided to increase the discount rate from the 8.4% E PRI used, to the 10.1% now considered to be the correct figure to use for 2009-10 costs. EL, I suggest you need to do some homework on the basis for the existing figures, before suggesting changes.
3. If you want me to spend time on your alternative calculations and assumptions, I’d urge you to lay out the assumptions, inputs, sources and calculations clearly so I can follow them. I am not going to waste time going through material that is not explained clearly and completely. At this point, I am convinced I’d be wasting my time.
EL
That is the capital cost for the OCGT generator plant. The fuel cost, including the gas delivery system, is included in the price of the gas. However, for the biofuel system we need to develop the infrastructure to get the fuel to the OCGT plants. So we need to include the capital cost to set up the biomass collection, transport, storage system, the conversion system to biofuel, the biofuels storage and distribution systems. On top oif that you have the O&M cost which is included in the fuel costs. I presume, since this is envisaged to be long term system, then something will have to be paid to the farmers for not just the collection and handling of biomass, but also for the degrading of the land and the fertilisers to partly replenish the nutrients removed.
Can you provide an authoritative cost estimate (from an authoritative, non partisan source) for the capital and O&M costs for the biofuel system which captures virtually all the residue from Australia’s grain crops – i.e. Western Australia’s too to feed eastern Australia’s electricity system.
Tell Western Australians about that idea 🙂
EL I’ll keep an eye out for more reports on German grass based biogas because I suspect the economics are bogus. Some questions
1) did they get a subsidy?
2) did they use diesel powered machines to harvest the grass?
3) how much effort does CO2 (typically 25%) removal take?
4) why sell the sludge when it could go back to the field?
5) when will a large (200+MW) OCGT plant run on biogas?
Call me cynical but everything about the German energy scene is suspect. I made an 80L digester based on grass clippings inoculated with cow manure with a mini gasometer for low pressure storage . After a few weeks I realised the smell and the work involved didn’t justify the weak gas output. it really helps if the sludge comes to you (eg via sewer or landfill) while oil and coal based energy are on tap. Any ‘green’ subsidy is the cherry on top.
IEA provides a bit more guidance on costs (current and future) for large scale biomass gasification plants in their following technical summary: “Biomass for Power Generation and CHP.” Current capital costs in the US are listed at $2,000-$3,000/kW, and future target costs at $1,000/kW. They reference a delivered biomass price of $3/GJ (which presumably factors in many collection and handling costs).
Making adjustment to input #4 on “best case” EDM cost model above for biogas generation ($931.6/kW AUS for plant cost, converted from 1,000/kW USD) one gets a final result: 164/MWh AUS (total system LCOE in 2030, relative to 2015 costs, and using lower EPRI cost estimate for TES storage, DRET-EPRI cost reduction factors for 2030, target IEA biomass plant and fuel cost, and ATSE recommended discount rate).
Here is relevant quote from IEA technical summary:
EL,’I’ve already referenced that document. Bits and pieces of data that are not put together in a coherent manner are meaningless to me.
The Gattan Institute Report gives a cost of about $5,500/kW for small plants of about 5 MW, To scale up to 30 MW plants, especially running on grain crop residues, has not been demonstrated as logistically feasible in Australia. We’d need 5000 x 5 MW plants, plus the storage for several years to get through the periods of drought and crop failures. We’d alos need massive over build.
I’d urge you to just apply sone engineering judgement to your ideas before putting them forward. The concept of the biomass generation proposed by EDM is ridiculous.
Did anyone notice the satelite map showing cloud cover over Australia yesterday. All the EDM proposed solar thermal power stations sites except Roma and Longreach were under cloud at the same time.
But, admittedly, the NEM’s wind farms were running at 50% capacity factor outside sun hours and up to 60% capacity at 2 pm (i.e wind at maxiimum at same time as solar at its maximum for the day):
http://windfarmperformance.info/
That sounds about right. “The number of biogas plants in Germany has increased by about 90% (2300 plants) in the last 5 years, with an average capacity of 500 kW compared to 60 kW in 1999. The introduction of economic incentives aimed at enhancing the deployment of renewable energies via the Renewable Energy Sources Act (EEG) has led to the proliferation of biogas production from large-scale plants (greater than 500 kW) organized in industrial parks, and with elaborate feedstock logistics” (page 185).
I don’t see where Grattan gives installed costs for gas/combustion turbine burning biogas at $5,500/kW. How expensive is it, after all, to put a PM filter on a OCGT plant? EPA gives installed costs for a CHP system to 40MW at $700-2,000/kW USD, and O&M costs at 0.08-0.11/kWh. The economics of larger biogas plants improve with size: less than $3,000/kW AUS (converted from euros) for plants exceeding 350 kW in European Biomass Association Roadmap.
I agree with you, the energy picture with biogas looks challenging until we get more experience with larger plants and have a better understanding of resource limits and availability in Australia. The links I have provided above suggest this is forthcoming (and may come up short for a full 100% biogas compliment and with a high degree of reliability). I don’t see any merit to adding fuel development costs to inflated capital cost for power plants (as you have done in your analysis). In fact, you have a fuel cost variable in your cost model, it seems reasonable to put these costs there (don’t you think)? The $10/GJ figure you have included seems reasonable to me based on other estimates I have seen. There’s a fairly good paper on grassland for biogas production Prochnow, et. al. 2009 perhaps worth a look for anybody who is interested.
The $5500/kWe presumably includes the cost of the biogas digester, the logistic infrastructure and assorted equipment. A large plant would also require a desulpurization unit to deal with hydrogen sulphide. Any good references on full cost breakdown for larger biogas units?
All three sources I have provided (one of which was previously used by Peter Lang and disregarded) provide total installed costs for digester, associated infrastructure, PM filtration, turbine, etc., for a combined biogas power plant or CHP plant. IEA ($2000-3000/kW USD in US), EPA ($700-2,000/kW USD for installed cost as CHP system to 40MW), and European Biomass Association reporting results for Germany (less than $3300/kW USD), and higher costs for Italy ($4250/kW USD). Frankly, it doesn’t make a lot of sense to build these things any bigger at this point in time. While the capital cost of doing so would be less, you’d be running up costs transporting feedstock, and you might as well put your agricultural waste in a landfill and just use more affordable natural gas. The research paper by Herr and Dunlop (2011) identifies about 17 areas in Australia where agricultural feedstock densities are sufficient for producing 500kt of stubble in less than 100 km radius, and numerous others where 200 km is needed. If Peter Lang wishes to continue to use the $5500/kWe AUS figure he should provide a source for doing so. Otherwise, using a high fuel cost value, as well as an unsupported capital cost for biogas plant, would lead some to disregard his final result as artificially high (for projected current costs of plants today).
If OCGT capital costs are $801/kW (DRET – EPRI, page 7-14), I have a hard time seeing how adding biogas production would result in an increase of 686% to the capital cost of the plant? If Lang would like to use a higher fuel cost than $10/GJ in his assessment for biogas, then he should provide a source for this as well.
EL,
You asked for the Grattan Institute reference. I don’t know why you can’ look it up yourself, especially since it has been discussed on at least two recent threads. But here it is again:
Gigure 8.6, http://www.grattan.edu.au/publications/125_energy__no_easy_choices_detail.pdf
The logistics for 30 MW plants, espeically using cereal crop stubble, are not demonstrated to be feasible in Australia.
The logistics to collect the stubble from all Australia’s grain crops to power the poposed gas turbines in Eastern Australia has certailny not been demonstrated to be viable.
Perhaps EL would like to attempt to provide costs for the proposed biofuel system to meet the following requirements:
– 24 GW power (but actally about 33 GW is needed to provide reliable power supply)
– equivalent of 290 PJ of gas fuel energy per year
– reliable supply of this energy through successive years of failed grain crops and decades of droughts
– biomass storage and biofuel storage sufficent to supply reliable electricity throughout all years and all seasons
– biofuel distribution system to the generating plants
– transmissions from the biofuel plants to the demand centres
I’d be seeking Capital, Fixed O&M, Variable O&M and fuel costs for the total proposed system of ‘gas turbines running on biofuels’. You need to explain how the crop residues will be collected.
At the moment I find your bits and pieces or unrelated and mostly irrelevant clippings as of little use for the many reasons I’ve already explained.
Came across this reflection on the above report on 100% renewable energy from my RSS feed, by Jean-Marc Desperrier on the Seeker blog. About the issues surrounding the 0.002% unavailability figure.
http://seekerblog.com/2012/03/02/comment-on-100-renewable-electricity-for-australia-response-to-lang-by-jean-marc-desperrier-jm_desp/
It appears that there are “devils in the detail”, as there always are.
EL and Ender have argued in numerous comments on this thread that the cost estimates I used are too high for Concentrating Solar Thermal (CST) parabolic trough, with 15 h storage, and the ‘gas turbines running on biofuels’. They have not presented alternative costs which are derived with a consistent methodology and assumptions across all the technologies. So the suggestions they have made so far cannot be applied. I remain satisfied that the costs used in Lang (2012) are the best available at this time for estimating the cost of the EDM-2100 simulated scenario and the variations of it.
EL also argued to use a different, lower, discount rate for the analyses. But it would be wrong to do this without fully understanding the basis of calculation of the discount rate used for the DRET LCOE figures and the proposed alternative (lower) discount rate, and presenting a valid argument to justify making the change. The discount rate was decided in AEMO and DRET sponsored stakeholder meetings, with Treasury and ABARE and other key players included, including the various renewable energy advocacy groups. I believe the figure is as good as we can get for now.
Despite me being satisfied the costs used are consistent between the technologies, are derived for Australia and are the best we have available, I have been looking at other approaches to these estimates. I’ve done some other estimates. These are not as well justified but they do show that even using other figures the rankings are the same, the conclusions are unchanged, and the revised figures make little difference to the absolute numbers.
First I substitute the US DOE/EIA (2011) capital and operating costs for biomass generation for the EPRI (2010), ACIL Tasman (2010), DRET (2010) costs. The new DOE/EIA costs are in 2009 US$ whereas the DRET costs are in 2009 A$. I have not made the necessary adjustments. Also, I do not know some of the inputs needed for calculating the DOE/EIA LCOE figures so I’ve adjusted the discount rate to calculate the correct LCOE figure for the capital and Fixed O&M component. What I’ve done is not strictly correct, but will do as a rough approximation.
The DOE/EIA LCOE for biomass generation assumes the fuel is wood and the plant runs at 83% capacity factor (average for its whole life) – so it is a base load plant. Conversely, the EDM analysis assumes the fuel is residue from Australia’s grain crops and the plants run at 13% capacity factor, i.e. strictly peaker plants, with most of the generation in winter. So almost a year of fuel must be stored for average years, and possibly many years of fuel must be stored to last through droughts. Therefore, clearly, the US costs cannot be applied to Australia. Therefore, I’d argue the Grattan Institute (2012) capital cost for 5 MW plants (Figure 8.6) is as good as we have available. I expect it is more likely to be too low than too high when all the costs are included.
But let’s do an exercise and apply the US capital and O&M costs, without making the conversion to 2009 A$, and see what difference it makes to the LCOE.
14. Biomass Bubbling Fluidised Bed (p14-1)
http://www.eia.gov/forecasts/aeo/assumptions/pdf/electricity.pdf
50 MW net.
Requires 2000 tonnes per day of wood (at 50 % moisture content)
Water consumption = (not stated but much higher than for a coal or nuclear plant)
Waste water is sent to the municipal waste water system or other wastewater delivery point – (i.e. the cost for such a facility is not included in the cost of the plant)
Adjacent substation nearby (i.e. not included in the costs)
2010 costs in 2009 US$
Capacity Factor = 83%
Capital cost = $3,724/kW (2009 US$) (excluding project finance costs)
Fixed O&M = $99.30/kW-year
Variable O&M = $6.94/MWh
Fuel = $35.36/MWh
Fuel = $2.50/GJ
Heat rate = 13,500 Btu/kWh
Thermal efficiency (HHV) = 25%
Points to note. These costs are for a wood burning, baseload plant. These costs cannot be compared with the cost for a system that is to operate on crop residue from Australia’s grain crops.
Compare LCOE at 83% and 13% capacity factor:
At 83% capacity factor, LCOE computes to US$111.6/MWh
At 13% capacity factor, LCOE computes to US$483.6/MWh
However, these are based on fuel cost of $2.5/GJ
This is less than half the cost of natural gas for electricity generation in eastern Australia. It is clearly much too low if we include the cost of the biomass system to produce, collect, transport, produce the biofuel, store the biofuel, distribute the biofuel and/or transmission cost from remote small power stations.
At a fuel cost of $10/GJ, the LCOE becomes:
At 83% capacity factor, LCOE computes to US$218.5/MWh
At 13% capacity factor, LCOE computes to US$590.4/MWh
However, all these figures are guesswork without a design basis and proper cost estimates for the proposed system.
Grattan Institute (2012) (p 8-9) states:
“For a 30 megawatt power plant at a 70% capacity factor the land area would be around 240,000 hectares and involve nearly 500 average sized wheat farms.”
For 24 GW power plant at 13% capacity factor the land area would be around 36,000,000 hectares and involve nearly 75,000 average sized wheat farms.
We can double or triple this figure to get us through long droughts and seasons of failed crops. We can also add the cost of storage to last for years of below average biofuel production. And we should add the cost of transport facilities used to move biomass from one location to another for when the crops succeed in one region but fail in another. We’d need roads, trucks, railway lines and rolling stock, all of which is to have sufficient capacity for the worst conditions but would be used rarely. The capital costs and the O&M costs must be included in the cost of the biofuel.
The costs just keep on increasing the more you think about the biomass idea.
Furthermore, Australia’s total area under grain crops is about 20,000,000 ha, so the EDM-2011 study needs twice as much land area under grain crop as we have. The 20,000 ha figure includes Western Australia’s grain crops. So we need to collect and transport all of Western Australia’s crop residue to eastern Australia to help to feed just half the EDM-2011’s demand for biofuel.
EL also argued we should extend the analysis to 2030 and use the projected costs for the various technologies for 2030. To extend the analysis to projection of the estimated costs in 2030 is fraught with uncertainties.
[…] Brave New Climate (e.g., Solar combined with wind power: a way to get rid of fossil fuels? AND 100% renewable electricity for Australia – the cost), so I won’t dwell on it here. Yet for The Economist to just leave the economic argument at […]