Guest Post by Ben Heard. Ben is Director of Adelaide-based advisory firm ThinkClimate Consulting, a Masters graduate of Monash University in Corporate Environmental Sustainability, and a member of the TIA Environmental and Sustainability Action Committee. After several years with major consulting firms, Ben founded ThinkClimate and has since assisted a range of government, private and not-for profit organisations to measure, manage and reduce their greenhouse gas emissions and move towards more sustainable operations. Ben publishes regular articles aimed at challenging thinking and perceptions related to climate change and sustainable energy at decarbonisesa.com.
Ed: This is a cross-post from Decarbonise SA.
————
This has got to stop, and it stops when people start taking a stand… The schism in environmentalism over nuclear power is now well underway. It is sad that the other side seem to have decided in their righteousness that they are allowed to play dirty and go after individuals, using the same cherry-picking abuse of science that is all to familiar in climate change denial.
I was saddened this week to be forwarded a hatchet job on my friend and collaborator, Professor Barry Brook, authored by Jim Green of Friends of the Earth (FoE). Saddened, but not surprised. FoE has form in this department, having deployed these guerrilla tactics before against James Lovelock when he became inconveniently persuasive on the subject of nuclear power. It would seem that it is now Barry’s turn.
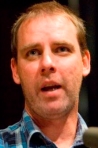
Jim Green, Australia’s anti-nuclear campaigner for Friends of the Earth
I have come to know Barry very well over the last 12 months. I know him well enough to know that he is both the last person who would ask for defending, and the most deserving of defence. So I offer this response to Green’s work. I really, dearly hope it will be read outside my circle of existing readers and supporters. I have some important things to say.
Green begins by getting some things really, really right. Namely, that Brook is highly qualified, highly regarded, extensively published, completely independent of the nuclear industry, and operating from a genuine concern about climate change. When you add to that the fact that he is highly influential, it becomes easy to understand why FoE have resorted to getting the hatchet out.
We are told Barry glibly believes “it’s nuclear power or it’s climate change”. This is an inaccurate and out-of-context portrayal of his position. It is a deeply considered and thoroughly researched position from a highly qualified scientist, the head of Climate Science at Adelaide University no less. It also happens to be a position that is largely shared by a long and growing list of prominent environmentalists (including the aforementioned Lovelock, James Hansen, George Monbiot and Mark Lynas) who have taken themselves through a similar process of critical examination of this problem as has Barry.
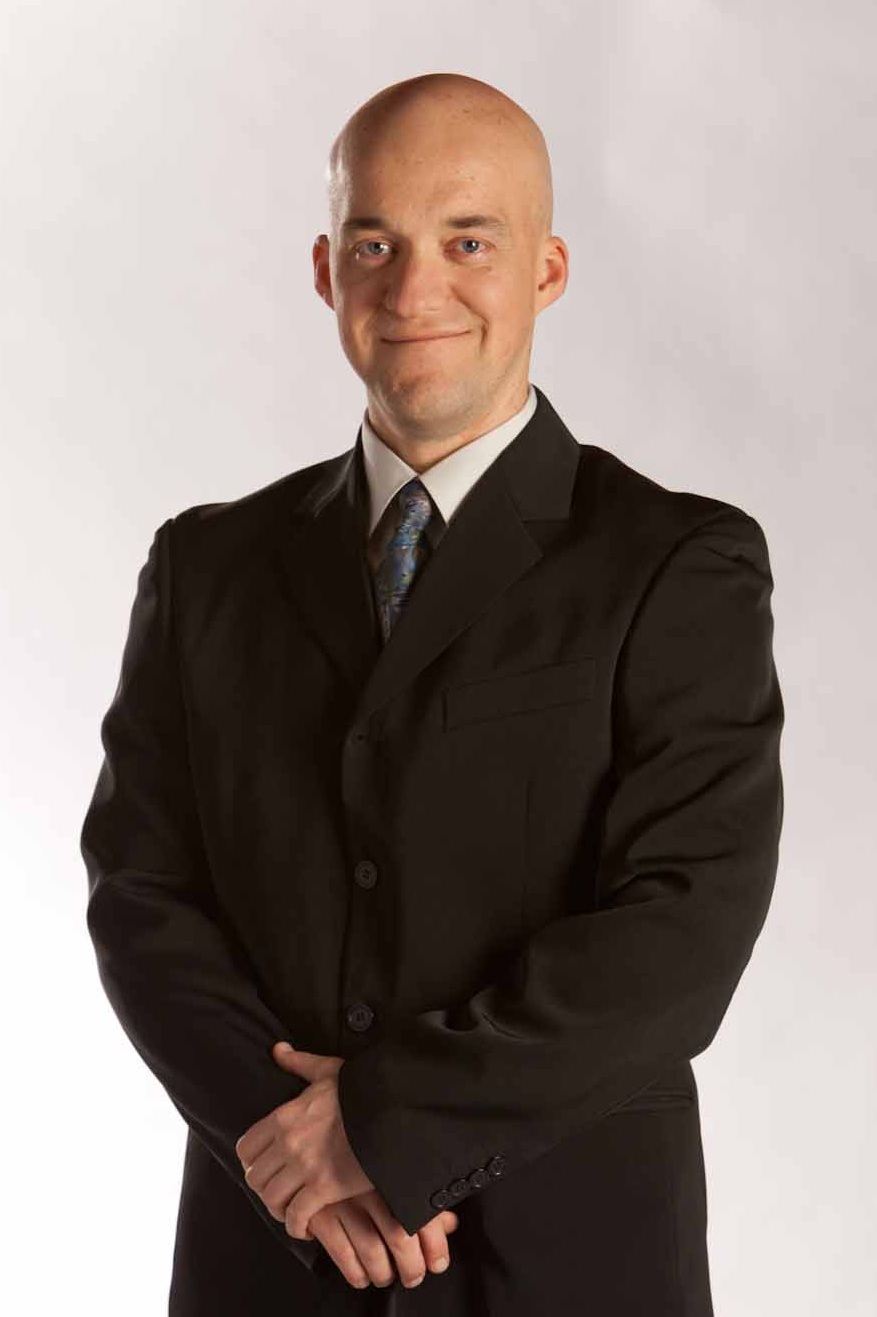
Barry Brook, Sir Hubert Wilkins Chair of Climate Change, Adelaide University. Prominent Australian nuclear advocate and founder of Brave New Climate
More times than I can recall, Barry has made the point that he does not care which technology does the job of rapid decarbonisation to avoid the worst effects of climate change. It is simply his well researched opinion that the central technology will need to be nuclear power or we will not succeed. Others are free to agree or disagree with him. But he states his case so cogently and robustly that every day more and more people are compelled to agree.
To suggest he is in error, Green refers to other, non-nuclear plans that supposedly demonstrate the redundancy of nuclear including a 2011 piece by Dr Mark Diesendorf of the University of NSW. I’m familiar with the Diesendorf study. I read both a critique of it and then a rebuttal from Diesendorf himself at this great site called Brave New Climate, run by a guy called Barry Brook. You see Barry (and therefore BNC) is not remotely concerned by robust debate on energy solutions. He positively encourages it, including running a very interesting and useful piece from none other than Jim Green! BNC is probably the best moderated and therefore most reliable place on the Australian web for robust, genuine debate.
Filed under: Hot News, Nuclear, Policy | 50 Comments »