As noted in my previous post on Integral Fast Reactors (IFR), Jim Green, from Friends of the Earth (FoE), has posted a critique of IFR.
Below, I (and others, names in square brackets), respond to his major points (in green): [BWB] = Barry W Brook, [TB] = Tom Blees, [GS] = George Stanford, [GLRC] = GLR Cowan. Furthermore, all of these arguments are also answered, in a variety of different ways, here, here, here, here and here. And of course in the book, Prescription for the Planet. So really, you could say that the below are just a small selection of the breadth of answers that are available to the interested reader.
As an aside, I note that I’ve now twice linked to the FoE critique via BraveNewClimate, so as to make readers aware that such critiques exist and to encourage people to read these and judge for themselves. I would hope that the FoE site similarly has the openness, and desire for balance, to link back to this rejoinder [Update: Jim from FoE has linked back to here].
—————————————-
What are IFRs? * non-existent reactors proposed to be fuelled by a metallic alloy of uranium and plutonium
[BWB] IFRs are sodium-cooled fast spectrum nuclear power stations with on-site pyroprocessing to recycle spent fuel. Fast spectrum power reactors exist — they are not some mythical ‘future tech’ like fusion reactors. Indeed, even sodium-cooled fast reactors (a type of Advanced Liquid Metal Reactor, ALMR), the type an IFR facility would likely use, already exist (others include lead- or gas-cooled). Metallic alloy fuels (uranium-plutonium-zirconium), operating within a reactor, existed, in the Experimental Breeder Reactor II at the Argonne National Laboratory. Just because they are not currently used in any operating nuclear power plant doesn’t mean they don’t (haven’t) existed. The only thing that doesn’t currently exist is the full systems design of the integrated IFR plant.
[GS] “Integral” refers to the fact that the fuel processing facility can be an integral part of the IFR plant.
…one or another largely undeveloped form of reprocessing/partitioning to separate transuranics (including plutonium) and actinides (long-lived waste)
[BWB] Transuranics are actinides — they are not separate things as the above implies. The process of pyroproccesing has already gone through significant technical development, but not commercial-scale demonstration. An excellent, colour-illustrated summary, from Scientific American magazine, is available (free download) here.
[GS] Transuranics are the elements beyond uranium – that is, their atomic number is 93 or greater: neptunium, plutonium, americium, curium and more. All of them are man-made elements, since they are so radioactive that the naturally created ones have long since decayed away in our little bit of the universe. They are also called higher actinides… An IFR plant will be a “sink” for plutonium: plutonium to be disposed of is shipped in, and there it is consumed, with on-site recycling as needed. Only trace amounts ever come out.
[TB] Yes, there will be more (unseparated) Pu involved in the entire process but once inside the door of the IFR it will never leave. With the sort of security and operational framework I propose in my book, it would be far easier to obtain Pu from another source such as a small research reactor. The bottom line is that while the IFR will be more proliferation-resistant than other designs, any time fissile material is used there should be some sort of oversight, even at small research reactors such as those found in many universities around the world. It would be far easier to produce isotopically favorable (for weapons) plutonium at one of them than to extract it from the fuel cycle at an IFR.
1. IFR fails the crucial weapons proliferation test. Anything that involves separating plutonium from spent fuel (even if Pu deliberately contaminated with unwanted radionuclides) increases the proliferation risks relative to leaving Pu in spent fuel… [and from a quoted reference] some elements of the technology still remain to be developed and demonstrated… The assessment of this fuel cycle should be an ongoing analysis that keeps up with the research rather than one based on the presumptions of either the advocates or the critics
[BWB] If you deploy IFRs in countries first in nuclear club countries — those that already possess, or are capable of making, nuclear weapons, then there is no additional proliferation risk. These countries already have a nuclear arsenal sufficient to wipe out humanity a few hundred times over. Building new IFR plants cannot meaningfully heighten this risk unless they are constructed in countries with no such capability. If this is done, it would require strong international oversight, as has been discussed elsewhere. Indeed, I’d argue that in consuming existing weapons-grade plutonium, the net effect of more IFRs is to lessen the overall risk of nuclear explosions.
But even without an international oversight organisions, we can reduce >95% of global greenhouse gas emissions by: (a) replacing electricity and transport energy with electricity from zero-carbon sources like IFR, deployed only in nuclear club countries, (b) halting deforestation in all countries (nuclear club and other), (c) massively scaling back agricultural emissions from fuel and ruminant/fertilizer sources, (d) providing non-nuclear-club countries with nuclear batteries, power via cross-border transmission lines, and boron or other metal fuels for vehicles, from IFR countries, (e) resolve the municipal solid was problem in all countries via plasma burners.
[GLRC] One can reduce the theoretical potential for power reactors to be involved in proliferation, but their actual history of involvement is zero, and so not subject to reduction. This potential remain like that of car engines to be made into multibarrel cannons: it could happen, and guns do proliferate, but never that way.
[TB] Pray tell, what is the problem with McFarlane’s statement: “The reactor … could be used for excess plutonium consumption or as a breeder if needed …” The fact that it can be used as a breeder is precisely why it would allow us to stop uranium mining, and the fact that it consumes excess plutonium is exactly what we want to do: get rid of separated plutonium and not separate it anymore.
[GS] A breeder is a reactor that is configured so as to produce more fissile material than it consumes. A fast reactor can be designed and operated to be either a net breeder or a net burner. A thermal reactor is a net burner of nuclear fuel, but – and this is very important – all thermal reactors are prolific breeders of plutonium. People often insist on calling IFRs breeders (originally, fast reactors were investigated because of their potential to breed), partly because of genuine confusion, and partly for the emotional impact, since “breeder” carries the subliminal connotation of runaway plutonium production. The central fact that those people are missing is that with IFRs you can choose not to breed plutonium, whereas with thermal reactors you make plutonium whether you want it or not.
– it is axiomatic that spent fuel provides a greater proliferation barrier than the proposed IFR mix of plutonium/actinides/fission products(?) since all those materials are in spent fuel along with other nasties.
– most importantly, none of the above makes a jot of difference if the proliferator has the capacity to further purify the plutonium in a reprocessing plant or a smaller ‘hot cell’ facility (which abound)
None of the above makes a jot of difference if the proliferator produces high-purity plutonium in the first place and if IFR is used as a plutonium breeder rather than a burner … no technical problems whatsoever using IFR to do just that (and the same applies to conventional reactors). So the WMD proliferation potential of IFR reactors (and conventional reactors) must weigh very heavily against them in any comparative assessment of energy options.
[TB] Green’s axiom is nothing of the sort. Spent LWR (light water reactor) fuel can be put through a PUREX (plutonium uranium extraction) process to extract virtually pure plutonium, though its isotopic composition will be far less than ideal for weapons. IFR fuel, on either end of the recycling process, would likewise have to be put through the PUREX process. Neither type of fuel can be handled without special remote handling equipment. So how does that make it axiomatic that spent fuel is a greater proliferation barrier?
In point of fact, anyone hoping to make a bomb from plutonium will likely try to obtain an isotopically more pure plutonium by creating it from U-238 (depleted uranium) at a small research reactor. To a great extent the proliferation threat of power reactors is overblown in light of this, but nevertheless proliferation resistance should always be a priority whenever fissile material is in circulation.
Green’s warning about IFRs being more dangerous in this regard is incorrect, since LWRs produce plutonium as well, and it’s in their spent fuel. Either way you need a PUREX process to extract the (isotopically inferior) plutonium. This whole issue is one of the most common misconceptions about the IFR system, and one of many under which Mr. Green is laboring.
I discuss at length in Prescription for the Planet how and where IFRs would be deployed in order to minimize proliferation risks.
As for breeding high-quality (I assume Green means weapons-grade) plutonium, virtually any reactor (including research reactors) can do that by wrapping a U-238 blanket around the core and letting it get bombarded with neutrons for a while, then removing it and extracting the Pu with the PUREX method. It requires relatively brief exposure, which is NOT what one would have in a reactor core operated for power purposes. Again, as I’ve pointed out here and in my book, fissile material should all be subject to rigorous international oversight. In P4TP I deal with just how to do that in some detail.
[GS] If their IFR plants were safeguarded, the material in the processing stream would be highly undesirable and their chances of diverting it undetected would be slim indeed. If not safeguarded, they could do what they could do with any other reactor — operate it on a special cycle to produce good quality weapons material. But in either case, most likely they would do what everyone else has done: construct a special production facility. Detecting such a clandestine facility is probably the main, immediate challenge facing international safeguards, and has nothing to do with whether a country has IFRs or LWRs.
2. They don’t exist. Long history of theoretically attractive reactors / fuel cycles which either haven’t been developed or have been highly problematic (e.g. breeders).
[BWB] See above for a comment showing that they (ALMR, pyroprocessing) do exist. Just saying they are fairytales won’t make the reality of them go away.
[GS] The problems with fast reactors (‘breeders’) have been non-fundamental. Examples:
– The Monju reactor was undamaged by the fire (rated 1 on a scale of 0 to 7, with 7 being the most serious accident), and has been kept shut down for political reasons. I think it has been given the go-ahead to start up.
– The EBR-II fast reactor worked flawlessly for many years.
– The Phenix fast reactor in France has been on-line for decades.
– The Superphenix reactor was shut down for political reasons, after it finally had its problems behind it and was working well.
– The Russian BN-600 has been working well for decades.
3. IFR envisages transmutation (bombarding some of the more problemtatic long-lived waste radionuclides to convert them to less problematic, shorter lived radionuclides) but this is problematic because i) it involves the proliferation risks associated with one or another form of reprocessing/partitioning of spent fuel into different streams (and thus facilitating plutonium separation, even if that is not envisaged during routine operations) and ii) the technology has been explored for decades but is still a long way from being mature.
[BWB] For answer to the proliferation risks, see above. Regarding the possiblity of reprocessing to purify plutonium:
“The diversion of nuclear fuel for the purpose of making bombs has been a concern, although presently the handling and destruction of nuclear weapons material is the primary issue. In the IFR, the nature of the fuel reprocessing is such that the fuel remains highly radioactive at all times. Fuel can only be handled in shielded cells or transported in casks weighing many tons. In addition, because the fuel recycle facility is located on-site, there is no transportation of nuclear which could create an opportunity for diversion. In any event, IFR fuel is not suitable for weapons without extensive processing in very expensive facilities. The potential also exists for the IFR to use weapons material for fuel, thus eliminating it, while producing electricity.”
[GS] Near-term, the IFR makes PUREX illegitimate and plutonium inaccessible. Long term, it relieves future generations of the responsibility to guard the plutonium mines, and of the risks of not guarding them adequately.
4. Safety risks associated with use of sodium coolant.
[BWB] The liquid sodium would be housed in a reactor pool with an inert argon overtopping atmosphere. The room in which the secondary sodium loop exchanged heat with the water loop would also be housed in an argon-filled room – a room separate to the reactor (see below Appendix for more information).
[GS] ALMRs use liquid sodium for cooling and heat transfer, which makes the system intrinsically safer than one that uses water. That is because the molten sodium runs at atmospheric pressure, which means that there is no internal pressure to cause the type of accident that has to be carefully designed against in an LWR: a massive pipe rupture followed by “blowdown” of the coolant. Also, sodium is not corrosive (on steel) like water is. Sodium can burn in air and react violently with water, and this of course requires prudent design, involving inert atmospheres and multiple barriers.
5. Brook says IFR will produce “very cheap” power. Good historical reasons to ignore such claims.
[BWB] I searched back through all my written material and comments, and cannot find where I said the quoted phrase ‘very cheap’. Regarding cost though, the standardised-design, factory-built, modular S-PRISM, is likely to be cost competitive or better than current generation nuclear power. I will detail this in a later post. The “good historical reasons” claim just doesn’t wash – there are good historical reasons to believe that renewables will never make up more than a small fraction of our total power generating capacity, but that’s hardly an argument against future expansion.
Further, from the UC Berkley FAQ:
“For a new power source to be viable, the cost of power must be competitive with today’s power systems. The proof of costs in any project only comes when full- sized systems are built and operated. Although no full-sized IFR plant has been built, several facts suggest that the IFR will be very economic. Costs of today’s nuclear plants are just slightly above that of coal as a national average. Several nuclear plants have operated with costs significantly below that of coal however. A new IFR should cost less than either a new nuclear (typical of today’s technology) or coal plant based on the following. The IFR does not require some of the complex systems that today’s reactors require. Examples include the low level radwaste cleanup station, the emergency core cooling system, and fewer control rod drives and control rods for comparable power. Because of the low pressure in the sodium systems, less steel is required for the plant piping and reactor vessel. There are studies that suggest that the reactor containment will be less massive. Other cost savings will be made because the IFR does not require the services of the Isotopic Separation Plants for fuel enrichment. Additional costs to the IFR include the integral fuel reprocessing capability, and a secondary sodium system (but the IFR fuel process costs are somewhat offset by the extremely low cost for raw fuel and the improved waste product). Some studies have been done which indicate that an IFR would be very economical and competitive to build, own, and operate, but the final proof of economics can only come in the construction and operation of a commercial sized plant.”
6. Ignoring the potential for renewables to produce baseload, intermediate- and peak-load power (see Mark Diesendorf’s paper on this topic at www.energyscience.org.au. Also ignoring the fact that 70-80+% of greenhouse emissions arise from sectors other than electricity generation – so Kirsch’s claim that IFR’s could be the “holy grail in the fight against global warming” is stupid.
[TB] Almost 80% of greenhouse gas emissions come from nuclear-capable countries anyway, so even if we just deployed them there we could make tremendous strides, though it would still be wise to create some sort of international oversight organization as I propose in the book.
[BWB] This is at best grossly disingenuous (not to mention insulting to call Kirsch stupid). You need to solve the electricity carbon problem to fix the vehicular fuels problem, space heating and embedded energy in building and manufactured goods, and Tom has a solution for MSW [municipal solid waste] also. About half of agricultural emissions can also be solved if you have a zero-carbon energy source. Then you just need to worry about the ruminant methane and carbon from deforestation. But the bottom line is, if you fix electricity, every else will quicktly start to fall into place.
If we don’t stop coal in places like China and India, we’re hosed, irrespective of what we might do in the US and Oz (and even if we could do with without advanced nuclear, which we very likely cannot). I do wonder, what is Jim Green’s plan is for replacing the 484 GW of coal-fired power stations already installed in China, and the further 200 or so plants in the planning or construction pipeline?
7. Still produces radioactive waste – albeit (in theory) a more manageable waste stream than conventional reactors. But to lessen the long-term hazards, the short-term public health, environmental and proliferation risks are increased through reprocessing, plutonium recycling etc. [Then cites MIT study, concerning how recycling transuranics will cost more than a once-through cycle]
[BWB] A 1 GW IFR power station would produced about 1 tonne of fission products a year. For comparison, a 1 GW coal-fired power station produces over 1 million tonnes. Plutonium (and other actinides) are indeed recycled in pyroprocessing, but Pu is never purified in an IFR, and would never leave the plant facility. Only the vitrified fission products would, which of course cannot be used in any nuclear explosive.
From the FAQ:
“Discussions on waste, nearly unlimited fuel supply, transportation, and a nearly diversion-proof fuel all hinge on the fuel type and the fuel reprocessing scheme. To describe the waste advantages, fuel reprocessing will first be described. Reprocessing of fuel is a key requirement of the IFR. However, IFR reprocessing is very different from processes which have been proposed or which are in use in other countries. Basically, reprocessing IFR fuel consists of two simple steps: 1. fission fragments are removed from the fuel, and 2. unused fuel is recovered, along with the transuranic elements (sometimes called actinides). Normally, the transuranic elements would go to the waste stream with the fission products, but in the IFR, they are kept with the fuel and sent back to the reactor to also serve as fuel. In the above description, note that the waste stream consists of only the fission products. The result is that instead of a waste that remains radioactive for many thousands of years, as would be the case if the transuranic elements were present, the radioactivity in the waste will decay to a value less than that of the original uranium ore in about 200 years. An additional advantage to the waste side of the IFR operation is that the IFR plant produces less low-level waste than today’s nuclear plants. The sodium coolant used in the IFR does not corrode the piping or structure, and, as a result, there are no radioactive corrosion products to remove from the primary system and send to a low-level radioactive waste repository. The fission product waste from an IFR type plant will amount to about 1700 pounds of waste per year for a plant of about 1000 megawatts electric output. This is in contrast to the waste from an equivalent coal plant of about 1,275,000 tons per year. These figures are for a plant that operates about 70 percent of the year.“
[TB] As for the MIT study that Green admiringly quotes, please refer to my book (pg 155–165) for a thorough trashing of same.
8. Brook says IFR reactors would be “safe from melt down” which is nonsense because technologies fail, well-intentioned humans err, and because the best laid plans can go awry if reactors are subject to sabotage or outside attack…
[BWB] The laws of physics say that this is not nonsense. For instance, the metal fuel pins’ composition is such that if they begin to overheat, the resulting expansion decreases their density to the point where the fission reaction simply shuts down. This is not speculation — it’s been tested and verified. I quote:
“The IFR gains safety advantages through a combination of metal fuel (an alloy of uranium, plutonium, and zirconium), and sodium cooling. By providing a fuel which readily conducts heat from the fuel to the coolant, and which operates at relatively low temperatures, the IFR takes maximum advantage of expansion of the coolant, fuel, and structure during off-normal events which increase temperatures. The expansion of the fuel and structure in an off-normal situation causes the system to shut down even without human operator intervention. In April of 1986, two special tests were performed on the Experimental Breeder Reactor II (EBR-II), in which the main primary cooling pumps were shut off with the reactor at full power (62.5 Megawatts, thermal) – By not allowing the normal shutdown systems to interfere, the reactor power dropped to near zero within about 300 seconds. No damage to the fuel or the reactor resulted. This test demonstrated that even with a loss of all electrical power and the capability to shut down the reactor using the normal systems, the reactor will simply shut down without danger or damage. The same day, this demonstration was followed by another important test. With the reactor again at full power, flow in the secondary cooling system was stopped. This test caused the temperature to increase, since there was nowhere for the reactor heat to go. As the primary (reactor) cooling system became hotter, the fuel, sodium coolant, and structure expanded, and the reactor shut down. This test showed that an IFR type reactor will shut down using inherent features such as thermal expansion, even if the ability to remove heat from the primary cooling system is lost. Events such as the loss of water to the steam system would cause a condition such as the test demonstrated. Another major feature of the IFR concept is that the reactor uses a coolant, sodium, which does not boil during normal operation nor even in overpower transients such as described above. This means that the coolant is not under significant pressure. When coolant is not under pressure, the reactor can be placed in a “pool” of coolant, contained in a double tank, so that there is no real possibility for a loss of coolant. Even if the normal pumps are lost, some coolant flow through the reactor occurs due to natural convection. The features described above allow for greater simplification of a nuclear plant, resulting in cost savings, greater ease in operation, and a safety system that relies on natural phenomenon that cannot be defeated by human error. “
[TB] Arguing that these reactors cannot be safe from meltdowns flies in the face of the laws of physics, which assure that very feature. Regarding terrorist attack, we can secure our airports chemical plants, etc, with not a lot of work, you can design these plants to be virtually impregnable by terrorists (e.g., burying the reactor building).
The new Gen III LWRs, though, are so far advanced as to merit their designation as a different generation. The probabilistic risk assessment of the ESBWR is astronomical, one core melt accident every 29 million reactor-years. Since we don’t have enough nuclear waste to load new IFRs quickly enough to meet the 2050 goal of zero emissions, the newest LWRs could be built to fill any gap that renewables and IFRs couldn’t fill and can be expected to perform safely. Their safety features are far beyond our current reactors by orders of magnitude.
Easy to make wild claims about non-existent reactors since such claims cannot be tested or disproved. As a nuclear industry representative has noted about non-existent reactor types: “We know that the paper-moderated, ink-cooled reactor is the safest of all. All kinds of unexpected problems may occur after a project has been launched.”
[TB] The assertion that such reactors don’t exist with the implication that they’re just fantasies on paper is bunk. BN-350 was operational for years starting in 1972. Phenix went online in 1973 and is still running. BN-600, still running and the most reliable nuclear reactor in Russia’s system. EBR-II ran for 30 years, FFTF at Hanford for many years too (I don’t recall exactly how many at the moment). Can’t make the fuel? They made thousands of fuel slugs at Argonne Labs over the years. Note the dates: 1972, 1973! And people say that something the French and Soviets built 36 years ago should take another 36 years for us to try? By the way, we will need plenty of desalination plants as our population continues to grow toward 9-10 billion. We don’t have 36 years to drag our feet.
Australian nuclear engineer Tony Wood notes that probabilistic risk assessment failed to anticipate the world’s worst reactor accident (Chernobyl) and the worst reactor accidents in the UK (Windscale) and the USA (Three Mile Island)… probabilistic risk assessment failed to anticipate the world’s worst reactor accident (Chernobyl)
[TB] While I seriously doubt that Green has access to such assessments about Chernobyl, our physicists in the USA made a conscious decision years earlier never to build a reactor designed like that because it was way too dangerous. Chernobyl was an accident waiting to happen. It has absolutely nothing to do with IFRs other than as a way to create a false equivalency and scare people.
[GLRC] Melting and blowing up are different, and no power station reactor built in any country that respected the advice Hungarian emigre Dr. Edward Teller gave in 1950 has ever had any way of blowing up. Chernobyl was a reactor explosion, not merely a meltdown. The chance of such an event in any Teller-compliant reactor is, as it has been since 1950, identically zero. (Parked cars are much heavier than parked bicycles or motorbikes, but their toppling-over risk is similarly not more, not the same, and not reduced by some factor; it is zero.)
—————————————-
NOTE: The above is a working draft only. I will update this post template as further relevant comments (including those posted below) come to hand. Please feel free to make suggestions below, or to email them to me.
—————————————-
Appendix
Critique of Chapter 6, “Generation IV Nuclear Reactors“, pg 115-130 in: Caldicott, H. (2006) Nuclear Power Is Not the Answer to Global Warming or Anything Else. Melbourne University Press. [Ch6 had input from Dr David Lochbaum]
By Tom Blees
P.122-3: Doesn’t differentiate between aqueous (MOX) and non-aqueous (pyroprocessing of metal fuel) reprocessing. You can’t conflate the two, they are very different.
P.123, last paragraph: breeders ARE fast reactors, just with a different fuel configuration to allow breeding of new fuel.
P.124: The design of the PRISM reactor makes it functionally impossible to lose coolant, and there is neither water nor air surrounding its containment, but rather argon. There is no egress below the level of the top of the reactor containment and with argon being heavier than air it’s there for the duration. The steam generator/heat exchanger is in a separate structure. The excursion events described here, with the ominous warning of a deadly radioactive cloud, are generically tossed out there with no consideration for whether they apply to any particular design of Gen IV reactor, and certainly not to the PRISM reactor, which has already gotten its preliminary seal of safety approval from the NRC with probabilistic risk assessments that exponentially exceed the safety of ANY other reactor design.
P. 125: “‘Closing’ the nuclear fuel cycle obviously will contribute to nuclear weapons proliferation.” What kind of ridiculous statement is that? What makes that obvious? Again, MOX and pyroprocessing are conflated, resulting in an “I’ll say anything I want and let the reader assume it applies to everything,” which of course is complete malarkey.
P. 126: 600 years is a stretch for strontium and cesium, which have about 30-year half-lives. Realistically it’s about half that. As for cost, a baseless assertion is made that fast reactors are twice as expensive as LWRs…”extraordinarily expensive,” in fact. Just look at the tone, how scare words are constantly interjected, as in “deadly fission products…deadly…dangerous.” And then comes the derision of the idea that these reactors are “sustainable,” when in fact the fuel is essentially limitless.
P. 126, last paragraph: Notice they forgot to put the word “Some” at the beginning of that paragraph.
Lochbaum’s testimony on P. 127:
– Why is it inappropriate to talk about Gen IV reactors before we dispose of all the waste, when Gen IV reactors would themselves dispose of it?
– One place Lochbaum and I can agree, that the NRC should be reformed. They should also be better funded so they can get their job done instead of operating on a shoestring.
– Again, all Gen IV designs are conflated here, convenient to Lochbaum’s purposes. His second sentence here absolutely does not apply to the PRISM reactor in any way whatsoever. His recommendation that new and untested materials, etc be tested in labs first is a ridiculous “Well, of course!” statement if ever there was one. Where does he think they develop these things?
Continuing his points on P. 128:
– Here he trots out the sodium volatility bogeyman, plus the misleading phrase “releases large quantities of radioisotopes.” Where are they released? They aren’t. And he talks about how the reprocessing involves the processing, transport, and storage of huge quantities of plutonium. Is he here conflating MOX and PUREX reprocessing with pyroprocessing? The latter, of course, never separates out plutonium and doesn’t require any transport of fuel except within the confines of the power plant.
– Again, conflation of MOX and pyroprocessing
– More griping about the Price-Anderson act, which automatically insures all reactors. He talks as if he expects private utility companies to waive coverage if they have safe plants, and that failing to do so (what businessman would?) betrays their dangerousness. I know there’s some Latin term for this, it’s a bit like a Post Hoc or maybe just an inductive fallacy. Pretty transparent, though, isn’t it?
P. 129: I won’t comment on the proliferation potential presented here because I think we need a better system anyway, which I propose in my book. As for the last paragraph, it’s more baseless assertions masquerading as facts.
Mercifully, P. 130: Having proved by unsupported assertion that reactors can’t be built soon enough to affect global warming, Caldicott/Lochbaum uses that “fact” to argue that it would be a grievous misallocation of scarce funds to put any money into nuclear, and just for good measure trots out the old saw about how the nuclear fuel cycle adds to global warming, which for IFRs is less true by far than it is of wind or solar construction projects since neither mining nor enrichment are necessary and nuke plants use 10-40 times less concrete and steel as comparable MW of wind turbine construction.
When finishing off, quoting Greenpeace, all Gen IV designs and intentions are again conflated.
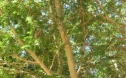
Filed under: Nuclear
I note that George Monbiot is also changing his tune on nuclear power — this was posted today:
http://www.guardian.co.uk/environment/georgemonbiot/2009/feb/20/george-monbiot-nuclear-climate
To quote:
“All I am seeking to do is to be clear about the opportunities and obstacles. I realise that this will provoke hostile responses from almost everyone – including my friends – but we do our cause no favours by obscuring the choices we face.”
And yet the text of the above indicates that he has not heard of IFR, as he still worries about the long-term storage problem.
China’s National Energy Administration recently announced an aim of an extra 60+ GW nuclear generation capacity over the next decade, which would bring nuclear’s contribution to about 10% of total current generation. Investment in renewables is proceeding apace.They are obviously aware of the coal problem; how long can they continue to extract 2.5 billion tonnes of it annually? Apparently almost half their rail capacity is taken up in shifting coal to power stations!
http://www.bloomberg.com/apps/news?pid=20601103&sid=avIwBKH8UfEk&refer=us
Feb. 17 (Bloomberg) — New nuclear power plants must be built to ensure that a strike by a commercial airplane won’t result in a radioactive release, the U.S. Nuclear Regulatory Commission said.
Any thought on what difference this makes for current boiling-water as compared to next-generation design costs?
One could as reasonably hope for “desire for balance” in an AGW denial site.
I — GRLC — didn’t write that very well, did I. This –
Given the huge potential of Geothermal energy, is a return to nuclear power really cost-effective considering the massive safety costs associated with it?
Here’s a response I’m sure you’ll get from the shock jocks: “If its so safe why do we need an international monitoring system? We don’t need one of those for solar thermal!” They will also point to cars overturned by cyclones as counterexamples to GLRC’s safety analysis of 4 wheeled vehicles and talk about the best laid plans of mice and men … etc. I’m now convinced that the risks associated IFR are smaller than the certainties associated with global warming, but the GW deniers are still a potent force so much will depend, in so far as public support is required, on the size of the population that is both skeptical of the risks of runaway climate change and also anti-nuke. I’m guessing that plenty of people who believe that we are changing the climate may not quite have caught up with the full implications of runaway change. The nuclear winter implications of a runaway nuclear exchange are far more familiar than the 6+ degree scenarios. The familiarity of images is critical to what people believe, making decisions based on evidence is pretty much a minority pasttime.
P.S. This is a gem of a post. Congratulations to all concerned.
A reminder, this is the source for most of the websites discussing how much uranium and thorium are being thrown away as coal is burned:
http://www.ornl.gov/ORNLReview/rev26-34/text/coalmain.html
Good stuff Barry!
Y’see, bleeders and sceptics DO have common ground!
Note: The post above has been update to include a critique of chapter 6 of Caldicott 2006, on Generation IV reactors (critique by Tom Blees).
reliable, cost effective, no waste problems, thousands of years of fuel supply….
… even if AGW IS a myth/beat-up/conspiracy this stuff just has to be built! I can’t think of a reason not to.
Sorry FoE -as a committed greenie I implore you to GET WITH THE PROGRAM!!!
A small note to Tom Blees :
Your arguments on how IFRs would be resistant to proliferation, more so than research reactors, is absolutely correct. We all know about it. But the high-priests of US foreign affairs & geopolitics have a slightly different view on non-proliferation.
For them, an unstable country / lunatic terrorist group getting hold of a nuclear bomb is not the definition of proliferation. (in spite of what they say).
For them, proliferation means another major power building up a nuclear arsenal large enough to threaten the USA with mutually assured destruction. Right now, only Russia has the capability of doing so. Both USA and Russia have nuclear arsenals large enough to destroy the entire planet several times over.
This huge weapons capability provides USA with a crucial leverage when talking with other countries on economic or geopolitical matters.
The strategic thinkers of the army etc think in terms of “how many nuclear hits can a country take and still survive”. The key word is nuclear deterrant. This is all bonkers of course. But this is the order of things. All this can be traced to the work of Albert Wohlstetter and his colleagues at the RAND corporation.
So research reactors which produce tiny amounts of high weapons grade Plutonium are not a “proliferation” risk, according to these guys. Somebody has to drive some sense into these people urgently.
Nick said @ 2, re. China: Apparently almost half their rail capacity is taken up in shifting coal to power stations!
Nick, you may be surprised to know that 44% of the rail freight in the USA is coal.
Hank @ 3, referring to airliner-proofing new nuclear plants: Any thought on what difference this makes for current boiling-water as compared to next-generation design costs?
Not much. The Westinghouse AP-1000 has an airliner crash-proof design that didn’t seem to add to the cost. Bear in mind that containment buildings even of the old style are really strong, so it was just a matter, in some cases, of modification. I suspect the new designs might even contain less concrete, but be constructed in such a way as to increase their resistance to such outside events.
OSO @ 5: Given the huge potential of Geothermal energy, is a return to nuclear power really cost-effective considering the massive safety costs associated with it?
I recently attended an energy conference with experts from all the various fields of alternative energy production. The geothermal guy wasn’t real encouraging. Nobody doubts that there’s enough heat under our feet to provide all the energy we need, if there was a way to tap it. But it’s not easy. There’s going to need to be some major technological breakthroughs. I hope they figure it out, that would be wonderful beyond words. But if we’re going to solve the climate crisis to the best of our ability, we have to resort to technologies that we know. Otherwise we’re betting our future on something that may never come to pass. Keep up the research on geothermal, for sure! Just don’t abandon solutions that rely on today’s technologies (IFRs included, which have been proven feasible).
I received an email from a friend saying you can’t connect a nuke of any kind to a coal fired power station because the turbines, boilers, etc are designed for different temperatures and pressures (now if P4TP only had an index I could check whether Tom covered this!). He says the only infrastructure that is saved is the transmission line end point. Comments please.
I was under the impression that Geothermal power is a mature technology as evidenced by power plants in New Zealand and Iceland.
The only technology really required is to dig the distance downwards. Once that is achieved (and underground digging is a fast developing technology) the sky is the limit.
OSO: you are thinking volcanic geothermal (e.g. based on hydrothermal surface vents), not hot dry rocks (HDR). The former is mature but can never scale up due to limited suitable places (e.g. NZ, Iceland), the latter, HDR, has a huge potential resource about 4-8 km below the surface, but requires a lot more R&D before its widespread viability is known. Digging down is only part of the problem — successful rock fracturing, water retention and cycling, avoiding localised cooling, mineralisation of fractures, etc. etc. Could all be possible to solve, but not ready yet and there are many places where it will never be applicable (Oz is potentially a very good place)
Geoff: Tom can answer further perhaps, but in essence, it depends on the temperature of the turbine used in the coal plant (e.g. standard pressurised vs supercritical boiler). In some case you will be able to use the same boiler and turbine, in others you will not, but can still use the cooling towers, basic plant infrastructure, transmission connections, etc.
I am also a great fan of George Monbiot, (see # 1) due to his rational approach and understanding of the urgency. He comments that 4 elements must be covered for nuclear to have his support.
1. Its total emissions – from mine to dump – are taken into account.
2. We know exactly how and where the waste is to be buried.
3. We know how much this will cost and who will pay.
4. There is a legal guarantee that no civil nuclear materials will be diverted for military purposes.
It seems that the IFR simplifies 1 and 2 (and maybe 4) to some considerable degree.
Ticking off number 3 would presumably involve a detailed design project for a commercial test project? How close and feasible is this?
[Ed: Point 3. is feasible — it’s called the S-PRISM design. I think any IFR advocate agrees that building the commercial demo is the single current priority]
Just checking, re the strength of the containment of existing plants, does that include the “ice condenser containment” design?
e.g. http://www.eia.doe.gov/cneaf/nuclear/page/at_a_glance/reactors/mcguire.html
On the Gen IV, is the fuel reprocessing included inside the aircraft-proof area? I worry more about a “sodium fire spreading through the plant” attack reaching a fuel reprocessing section than about someone methodically stealing plutonium.
On replacing coal burners with fission — there ought to be a table somewhere with the design temperature of all the coal plants in use.
That would be a shopping list for placement of new-gen heat sources. I gather it’d be the old ones burning at low enough temps that a fission source could replace them straightforwardly.
That would be a good thing. It might also satisfy the coal companies by removing their worst polluting and least efficient committed uses.
Geoff @ 14: I received an email from a friend saying you can’t connect a nuke of any kind to a coal fired power station because the turbines, boilers, etc are designed for different temperatures and pressures (now if P4TP only had an index I could check whether Tom covered this!).
The heat exchanger and reactor would replace the one from the coal plant. After that, as long as the turbine is sized to the reactor(s) that generate the steam, it’s good to go. If not, you can use an off-the-shelf turbine. Obviously the whole cooling system (towers or whatever) is also sized to the turbine capacity and its steam loop and condenser. That’s one reason why PRISMs are nice, because they’re relatively small (360MWe) and can be clustered so as to match up pretty well with most coal power plants as needed.
Sorry about the lack of an index! I was trying to publish quickly and also didn’t have the money for it. You know, the struggling author thing…
OSO @ 15: Au contraire, my friend. Geothermal is done now in those relatively rare locations where it’s readily available. There are major problems associated with it, not the least of which is channeling, i.e. the water tends to form channels from the water input to the steam output side, quickly cooling the channel area until pretty soon you’ve got cold water in and warm water out. It is definitely NOT a mature technology. If it were these conversations (and my book) would be moot. Oh, I see Barry already dealt with both of these a bit. Gotta get up pretty early to beat Barry to the punch.
Hank @ 17: The pyroprocessing would be done in a hot cell separate from the sodium areas, and in fact the hot cell could (and likely would, from a practical standpoint) be built underground, or in areas where high water tables might make that impractical it could be simply heaped over with earth after its built, making it essentially an underground structure. Just have to use enough earth to stop a plane. Dirt’s dirt-cheap.
For those in SF Bay Area, see (public) energy seminar @ Stanford Wed Feb 25, 4:15-5:15:
Jacques Bouchard, former head of the Nuclear Energy Division of Commissariat a L’Energie Atomique in France
Can Nuclear Energy be a Sustainable Contribution to Address the Climate Change Concerns? The French Experience
Google: jacques bouchard generation iv
for why his talk might be relevant to this topic.
Thanks a lot for that info, John. I will definitely attend, being only about 1.5 hours away from Stanford. I’ll welcome the chance to discuss the timelines with Bouchard. His ultra-conservative 2030+ timelines don’t differentiate the far-from-ready Gen IV technologies from the PRISM, which could be started to build next week, according to the leading experts on that technology who I’ve worked with for years. Notice his estimates of cost of about $1,000/kW. I’ll take ten! Thousand, that is!
One thing about the French and nuclear: AREVA is based on making lots of money building LWRs with oxide fuel and MOX recycling, all inferior to the IFR system in virtually every respect. I don’t know how dedicated Mr. Bouchard is to his home country’s industry, but he certainly doesn’t seem to be in any hurry. It should be an interesting talk. I do hope he allows time for discussion afterwards. BTW, it wouldn’t surprise me if he’s unaware of GE’s capabilities and possible timelines regarding the PRISM, since GE is AREVA’s competitor. And it’s a very serious competition.
They usually allow time for questions, sit near front, there are usually more questions than time.
By a truly bizarre coincidence, my wife had had for many years a consulting business named Areva International, with a domain = http://www.areva.com.
When Areva was formed, she started getting lots of email misdirected to that, rather than arevagroup.com, and ended up selling it to them.
It would also be interesting to know Anne Lauvergeon’s opinions on all this.
I’ve talked to her about it, actually, and will surely do so a lot more in the future. Though AREVA’s default position on IFRs is generally negative, she personally didn’t say a peep against it even as she listened to presentations that at some points fairly clearly stated that IFRs were superior to MOX and oxide fuel systems. Anne is smart enough (VERY smart, to be sure) to realize that if we work together to overcome the resistance to nuclear power in general and begin to build lots of reactors, AREVA is in a better position than just about anybody to make hay off it. It doesn’t really matter which flavor of reactor we’d be building, they’re fully capable of fabricating the components and are upgrading their manufacturing capacity while others are standing still. Rather than feeding a spirit of negative competition, I hope we can soon see all the major vendors building IFR components complementarily for the benefit of all. Granted, AREVA would understandably probably prefer to just move ahead with the systems they currently build, but if the tide starts turning to IFRs they’ll be on the crest of that wave too, you can bet on it.
Tom and/or John, do give us a brief update after the Bouchard talk! I note that GIF have the SFR down as being earlier than 2030:
“Owing to the significant past experience accumulated with sodium cooled reactors in several countries, the deployment of SFR systems is targeted for 2020.”
http://www.gen-4.org/PDFs/annual_report2007.pdf
any comment on the feasibility of this idea…
http://www.cosmosmagazine.com/news/2589/fission-fusion-hybrids-could-mop-nuclear-waste
thanks
As promised I am not commenting on the IFR.
I have to see for myself how Tom treats this.
Some things however I have observed in my research before I get the book:
1. If nuclear could be made into international non-profit why not make all energy non-profit as well. Then we could ration the remaining oil and gas in a rational manner for all humanity. Also then all renewables would be also released from having to make a profit so they would be far more competitive.
2. Boron cars are not even on the drawing board. I can find only one paper describing them. A far better path would be zinc-air fuel cells that have been trialled.
http://www.electric-fuel.com/ev/index.shtml
There’s more than one way to boil water (and with wind power you don’t even have to boil water). And I wouldn’t choose the most complicated especially when that choice increases the likelihood of cancer, serious injury or death, amongst other external costs.
I would choose Glass + Concrete + Steel — which happen to be amongst the most common materials and inputs available for industrialised economies today to boil water.
I have details here of commercially installed and operating today — with more plants being built Concentrating Solar Thermal — I have real data here on Land Use and materials use.
Firstly I recommend these two video’s
PS10 Abengoa Spain – Solar Thermal with Storage
Solar Tres Spain 74% Capacity Factor 6482 out of 8760 hours of generation per annum.
http://www.youtube.com/watch?v=z3ypQo-gzf0 (48 secs -> 3 mins)
Andasol1 and Andasol 2 trough plants at 50MW each capacity factor 55% with 7.5 hours storage are already a reality. The mixture, 28.5 tonnes of 40% potassium nitrate and 60% sodium nitrate sits inside two 36 metre diameter, 14 metre high storage tanks. A cold tank and a hot tank.
No you do not have to increase the “nameplate” capacity to increase the capacity factor. Capacity factor in the energy industry is always derived from the maximum electrical output which is measured at the generators interface to the grid. What you are doing to achieve 74% or higher capacity factors is increasing the size of the mirror field and adding storage. The thermal losses calculation (which is less than 10% for troughs and even better approaching 7% for higher temperature systems such as towers) is made up for with a slightly increased mirror field size.
What are the specifications of Ausra’s Carrizo Plain CA plant..
258 hectares or 640 acres nameplate 177MW plant (177*.25)*24*365 = 387.630GWh
How much electricity is used in Victoria?
Victorian Electricity consumtion 54,000GWh
**How many Plants of this size do we need?
54,000 / 387.63 = 139.3
Nameplate of plants combined 24,715MW
**How much land would these plants take?
258 hectares * 139.3 35,939 hectares
**How much land do current latrobe valley power assests occupy?
25,000 hectares — Loy Yang Open Cut alone is on 6000 hectares of land.
**How much land is there in Victoria?
22 741 600 hectares
**What Percentage of land would it take to do this with Ausra plant?
0.15% of Victoria
How much materials would all of Victoria’s power provided by CSP take?
Although material usage is lower now than when this paper was written this gives you an idea of the amount of glass steel and concrete required.
http://solar1.mech.unsw.edu.au/glm/papers/Mills_projectproposal_newcastle.pdf
Steel per MWe = 44 tonnes *24715MWe = 1,087,460 tonnes steel
Glass per MWe = 5.4 tonnes * 24715MWe = 133,461 tonnes glass
Concrete per MWe = 64 tonnes *24715MWe = 1581760 tonnes concrete
Concrete
http://www.concrete.net.au/industry/VIC_concrete.php
In 2006 Victoria produced 13.2Million tonnes of Concrete in order to build enough ausra solar thermal to power Victoria we would need 158,716 tonnes of concrete per year or 1.19% of annual production of Victoria. This would be the same percentage of concrete production australia wide to build enough Ausra CSP plant to power Australia
Steel
Bluescope manufactures 5Million Tonnes of Steel annually
Onesteel manufacturers 1.2Million Tonnes of Steel Annually
http://en.wikipedia.org/wiki/OneSteel
http://en.wikipedia.org/wiki/BlueScope_Steel
For Victoria we will need 108,746 tonnes of steel per annum to build out the Ausra plant or about 1.75% of annual steel production.. For the rest of australia mulitply this figure by 4. We would require about 7% of Annual Steel production.
We are taking 1million tonnes of steel production off line this week because of the economic down turn. And this is enough steel to power Victoria with Concentrating Solar Thermal.
http://www.theaustralian.news.com.au/story/0,24897,25092915-643,00.html
Glass
PIlkington produces 215,000tonnes per annum around 30% of the market so in other words per annum we are producing 645,000tonnes
For Victoria we will need 13,346 tonnes per year over 10 years or 2.07% of Australian annual production. Victoria is roughly 25% of Australia’s energy demand so to do the rest of the country would take 8% of annual glass production.
I have got the real life figures for power towers here — to supersede all the misinformation that has been presented previously.. Here is data for 2 of 9 international power tower companies. It is also worthy to note that efficiencies will increase by 50% in many systems reducing the land required by 1/3. This is because a number of companies in Dish and Tower systems are putting Brayton Cycles in front of a Rankine cycle, initially using air as a working fluid at upto 1000C then the waste heat at around 400 runs through a rankine cycle. yielding 27% versus 18% off the mirrors for a regular system as quoted in these figures above and below. (to electrical)
http://www.abengoasolar.com/sites/solar/en/technologies/concentrated_solar_power/power_tower/index.html
95 hectares 20MW plant 25% Capacity factor 43.800GWh
54,000GWh per annum Victoria / 43.8 = 1232.8 power plants
Land Area 117,040 hectares or 0.51% of Victoria’s land out of 22,741,600
http://www.esolar.com/
64 hectares 46MW plant 25% capacity factor 100.74GWh
54,000GWh per annum Victoria / 100.74 = 536 power plants
Land area 34,306 hectares or 0.15% of Victoria’s land (22,741,600)
Also on water use.. an Ausra solar plant uses
0.18 litres per MWh as compared to 2litres per MWh for a Victorian Brown Coal plant.
Matt #27
As you quite correctly state, the 74% ‘capacity factor’ of the solar power towers is obtained by using molten storage, achieved by adding to the size of your mirror field. What are the implications of this?
If you had a CSP plant with a peak capacity of 1000 GW and a 20% capacity factor (no molten storage), then it would generate 200 MW, or about 1,750 GWh/year. It would generate close to peak capacity during the day when it was sunny, in the summer, about half of peak in winter days, and zero output at night. Hence the 20% or so average overall.
Over the course of the year, let’s say it (on average) generates 1000 MW for 2 hours, 700 MW for 2 hours, 500 MW for 2 hours and 200 MW for 2 hours, and then nothing at night. That would be 4,800 MWh per day, which, averaged over 24 hours, gives a capacity factor of 20%. How would we get this capacity factor up to 74% via storage?
We’d need to build more mirrors, as we said above. We are seeking 17,760 MWh per day. So we’d need to build 17,760/4,800 = 3.7. Then there are conversion losses for thermal storage — let’s use your figure of 7%. This raises our mirror factor to 3.98. So we need to install about 4000 MW of peak capacity. As is clear, we haven’t made that baseline 20% capacity factor go away. We’ve just changed our definition by increasing the mirror field and using a different reference frame (net power vs nameplate).
So you can define capacity any way you like, but it doesn’t avoid the fact that to yield that consistent electrical delivery from CSP, you must build a lot more mirrors and storage. This has a cost in terms of land area, materialsand labour for manufacturing and construction, fuel and general logistics in getting materials to desert sites, water required for regular power washing of mirrors, etc. All achieveable, as you rightly point out, but the cost rises in proportion to the capacity factor rise.
So if it cost you $US 1,500 per peak KW in 2020 to install such a system, then in terms of KW delivered, the actual cost is going to be 4 times higher, i.e. $6,000/KW. Mills and Morgan are currently reporting $ 3,000, which takes the delivered figure to $12,000/KW (i.e. $12 billion per GW). Bottom line: increasing capacity factor axiomatically increases price and logistical issues in installation and maintenance.
Now I absolutely believe that CSP peak prices will continue to fall thanks to the benefits of scale-up and experience (the same will be true of standardized-design, factory-built, modular IFR units, I might add). But right now, our best reference on price is what’s actually been done (‘proven up’), not what is claimed or projected (the same is true for nuclear power, of course!).
For decent-scale power towers, the best example we have to look to so far is Solar Tres, as you said: http://www.solarpaces.org/Tasks/Task1/Solar_Tres.htm
This is a 17 MW solar tower in Spain with “74% capacity”. The cost is €196 million. Based on their reported 111 GWh/yr yield for the 17 MW plant, this works out to a cool €15.5 billion per GW installed.
You mentioned the Ausra Carrizo 177MW (peak) plant, which is discussed here (along with Kimberlina):
http://ecoworld.com/blog/2008/11/11/ausras-kimberlina-solar-thermal/
They say: “Ausra’s planned Carrizo plant, at full scale, projects a somewhat lower 206 megawatts per square mile in full sun, but that still equates to 370,000 megawatt-hours per square mile per year.”
370,000/8760 = 42 MW for 206 MW installed, so a capacity factor of 20%, not the 25% you state above. Cost estimate is difficult because it isn’t finished, but the estimate is around $500 million, i.e. $14 billion per GW.
Regarding your steel, glass and concrete estimates. The paper you derive them from is a 5 MWe peak capacity (linear fresnel) plant. The annual capacity factor of this plant is 17%. So to derive 1 MWe, you need to multiple your above figures by 5.88. That is:
Steel/MWe (generated) = 259 tonnes, Glass = 32 tonnes, Concrete = 527 tonnes (based on 1.4 t/m3).
This may well be what you meant above – your later multiplication by 24 GW certainly does imply this, although with a capacity factor of 17% rather than 25%, you need a little more material than you quote.
Now I agree with you that the land area of desert that must be acquired (where you would put these things), and the volume of steel/glass/concrete we must manufacture for scale-up, is not the problem with CSP, if we treat the zero-carbon energy issue with sufficient seriousness — these are not show stoppers.
However, it still leaves a number of questions (there are more, but this is enough for now):
1. Given the oversizing required to increase capacity factors, will CSP ever be a cost-competitive option compared to other low/zero carbon energy alternatives (including nuclear)?
2. What do you do when you have a few cloudy days in a row, especially in winter when generating capacity is at half the summer insolation? How much extra thermal storage do you build in to your system to stop extended blackouts?
3. Do you engineer it to deliver the 6 GW average power demand from Victoria in winter, which means it’s on average delivering twice what’s needed in summer? If so, what do you do with the extra power — dump it, or store it chemically?
4. 34,306 ha = 343 km2 (or 1117 km2 for the other estimate). Installing this in 10 years requires covering between 94,000 to 306,000 m2 of desert every single day over that period, to meet Victoria’s average energy requirement. Do you consider this, or even half this (if the other part was to come from wind), to be logistically feasible? [For comparison, the 64 MW Nevada Solar One project took 16 months to cover 1.6 km2, or about 3,370 m2 per day]
5. How much extra embodied emissions are involved in rail freighting, and then trucking to site, the steel, glass and concrete?
6. How much water is required to power clean 343/1117 km2 of mirror field every 2 weeks? (this is beyond the water used in the turbines). How do you get this quantity of water to the desert?
Also, could you kindly point me towards the ‘all the misinformation that has been presented previously’, so I can once again check my sources?
Ender, regarding 2.: Correct, boron cars are nowhere near as developed as IFR, but they certainly deserve some serious R&D to evaluate feasibility and commercial viability (irrespective of whether the power needed for boron reduction comes from IFRs or other sources such as renewables, or both).
There’s no doubt that it’s a great idea and many folks suspect it could work well, but it might end up being aluminium- or zinc-powered cars, or it might not turn out to be practical and we have to go for an entire fleet of BEVs.
If the one paper on boron cars found by Gloor was the one of mine that I have made easy to find, these words in it,
explain why I disagree with some halfway approaches that are now being taken or discussed. It is possible to react aluminum with water, or boron with water, and get hydrogen, but this is a wasteful use of the energy in the B or Al, and it gives you hydrogen, a fuel that burns readily or explosively in air. You don’t want hydrogen.
It is true that zinc-air power for vehicles has a track record. The “Metallic Power” outfit I mentioned there is long gone, and zinc-air power looks like a good bet to deprive governments of $0 in fossil-derived motor fuel sales per decade for decades to come.
(I see I first linked those notes from a 2002 discussion titled, “What’s the fuel cell holdup?”! In the linked charts, more favorable data have darker cell backgrounds.)
(How fire can be domesticated)
Some comments here by me on Obama’s energy plan:
http://www.sciam.com/article.cfm?id=obama-wins-praise-for-ren
Dear Barry,
The point where our communication is stuck is at the annual demand. You are assuming I have not provisioned enough mirror field.. here you are wrong
I acknowledge that the mirror field to deliver 75% capacity factor for a given turbine genset is much bigger in fact roughly 3x + an additional 10% premium for storage on the night time component of that.
BUT I had already taken that into account in my calculation. Because I had based my calculation on GWh delivered annually. And had sized the mirror field accordingly.
ie here..
What are the specifications of Ausra’s Carrizo Plain CA plant..
258 hectares with a nameplate of 177MW which is the maximum output of this plant.
Assuming a low light conditions (like a country other than Australia) I will adjust the figure for 20% (Australia Mildura / Moree / WA -> 25%)
Energy Generated per year = (177*.20)*24*365 = 310.1GWh
Assuming a 20% capacity factor.
Victorian electricity consumption in 2008 was 54,000GWh
How many plants of this size do we need?
Victorian Consumption (54,000GWh) divided by The amount of power delivered by each plant annually (310.1GWh) = the number of plants of Carrizo size that we will need to build in order to supply the Victorian grid with it’s annual demand (based on 2008)
So the figures above stand, and we can progress this conversation, to look at any other issues.
I can also add a 10% premium to 2/3 of the mirror field size to allow for storage overheads for the 2/3 that are delivered when there is no / low sun?
Matt, yes, I know you took those considerations into account, and my list of 6 questions were framed on this basis. My point, in stepping through the ‘capacity factor’ calculations, was to illustrate the cost/size-up implications of this for CSP, which set the context for my 6 concluding questions re: the logistical feasibility and cost competitiveness of CSP [or other variable sources such as wind] vs other zero carbon sources of energy such as nuclear.
Dear Barry,
If you know that then we need to know we’re on the same page so that we know for a theoretical solar scenario (Later we will discuss the issues of winter and cloud cover etc)
So we know we’re on the same page we need equivalent mirror field of 174 Carrizo Plants (using your incorrect for Australia 20% capacity factor) (we only need 1/3 the turbo gen sets though) but we do need 2/3 storage capacity for 12-19hours.
Therefore For the mirror fields in concrete steel and glass terms we require
Steel per MWe = 44 tonnes *30798MW nameplate = 1,355,112 tonnes steel
(1.1 Million tonnes of steel – the amount just turned off at Blast Furnace Number 7 Port Kembla)
Glass per MWe = 5.4 tonnes * 30798MW nameplate = 166,309 tonnes glass
Concrete per MWe = 64 tonnes *30798MW nameplate = 1971072 tonnes concrete
I am going to contact the peak bodies or leading companies for concrete and glass and see how much drop they’ve had in production/sales since the financial crisis kicked in.
My hypothesis is that the slack in the factories could almost totally be taken up building out these plants.
once we’ve confirmed we’re on the same page I’m happy to move to discussing the next point.
Matt
For the previous post.. The agreement I am looking for .. is the amount of Concrete Glass and Steel to supply 54,000GWh electrical of Solar Thermal ie the equivalent consumption (2008 of electricity in Victoria)
I agree with those concrete, glass and steel figures.
Barry and Matthew – “the logistical feasibility and cost competitiveness of CSP [or other variable sources such as wind] vs other zero carbon sources of energy such as nuclear.”
Some latest figures for nuclear (I assume we are still talking GenIII)
http://www.world-nuclear.org/info/inf02.html
“Based on this study, Florida Power & Light in February 2008 released projected figures for two new AP1000 reactors at its proposed Turkey Point site. These took into account increases of some 50% in material, equipment and labour since 2004. The new figures for overnight capital cost ranged from $2444 to $3582 /kW, or when grossed up to include cooling towers, site works, land costs, transmission costs and risk management, the total cost came to $3108 to $4540 per kilowatt. Adding in finance charges almost doubled the overall figures at $5780 to $8071 /kW. FPL said that alternatives to nuclear for the plant were not economically attractive.
In March 2008 Progress Energy announced that its two new Westinghouse AP1000 units on a greenfield site in Florida would cost it about $14 billion, including land, plant components, cooling towers, financing costs, licence application, regulatory fees, initial fuel for two units, owner’s costs, insurance and taxes, escalation and contingencies. If built within 18 months of each other, the cost for the first would be $5144 per kilowatt and the second $3376/kW (average $4260/kW) – total $9.4 billion. Interest adds about one third to the combined figure – $3.2 billion, and infrastructure – notably 320 km of transmission lines – about another $3 billion. The units are expected on line in 2016 and 2017 and are expected to save customers some $930 million per year relative to natural gas-fired generation. Progress emphasised that nuclear would be more cost-effective than alternatives. At the end of December 2008 the company signed an engineering, procurement and construction contract for $7.65 billion ($3462/kW), of an overall project cost of about $14 billion, including land, inflation, site preparation, licensing, financing costs and fuel.
In May 2008 South Carolina Electric and Gas Co. and Santee Cooper locked in the price and schedule of new reactors for their Summer plant in South Carolina at $9.8 billion. The EPC contract for completing two 1,117-MW AP1000s is with Westinghouse and the Shaw Group. Beyond the cost of the actual plants, the figure includes forecast inflation and owners’ costs for site preparation, contingencies and project financing. The units are expected to be in commercial operation in 2016 and 2019.
In November 2008 Duke Energy Carolinas raised the cost estimate for its Lee plant (2 x 1117 MWe AP1000) to $11 billion, excluding finance and inflation, but apparently including other owners costs.
In November 2008 TVA updated its estimates for Bellefonte units 3 & 4 for which it had submitted a COL application for twin AP1000 reactors, total 2234 MWe. It said that overnight capital cost estimates ranged from $2516 to $4649/kW for a combined construction cost of $5.6 to 10.4 billion. Total cost to the owners would be $9.9 to $17.5 billion.”
So nuclear in 2008 can have costs from a low of $2516/kW to a high when interest is figured in of $8071/kw. Also these are US prices where a nuclear infrastructure exists. In Australia at least for the first reactors there would be a premium because everything except sand, cement and perhaps mild steel, would have to come from overseas.
Also not all the CSP plants would have to have storage as the peaks when it is needed would be quite sufficient for perhaps 30% of the CSP plants as we do not need as much supply at night.
For the purposes of the capital cost comparison you have to use cost\nameplate capacity so the Ausra plant is 500 000 000\177 000 ~ $2800 /kW which is at the lower end of the nuclear capital cost. To get to the upper bound of recent nuclear costs you would have to triple the cost of the solar plant and this would encompass at least a 3 times oversize mirror field plus storage.
So at the ballpark capital cost stage of estimation, that does not include capacity factors, a CSP solution in Australia could well be cheaper than nuclear. Again you would need some more numbers to estimate the cost/kWh because this calculation does include capacity factors.
However more realistically we would have some of the scenerios detailed in:
http://www.wwf.org.au/publications/clean_energy_future_report/
Ender/Barry/Matthew: I’ve raised this before, but Matthew wasn’t around then. In Australia, an additional factor favours solar. We can use local dollars and we don’t (shouldn’t) need foreign expertise, but with nuclear, we need foreign dollars, often raised by selling coal/beef/aluminium. That factor is hard to quantify but real nonetheless.
Ender, I’ve pointed out numerous times the brokenness of the corporate/regulatory/government hodge-podge regarding nuclear in the USA, both here on this site and in my book. Look at the Japanese experience to see what CAN be done. $1.4 billion USD/GW. Those are the most recent plants built, 2 of them. Why is it taboo to use that real-world data instead of screwy projections from rip-off private utilities in the USA? You get no argument from me that the USA is totally screwed up when it comes to nuclear, as Oz may be for all I know. What the Japanese experience proves beyond a shadow of a doubt is that modern nuclear power systems are not inherently expensive. They CAN be built very economically, probably even cheaper than the ABWRs in Japan because the more advanced designs are even simpler and smaller.
I’m not even going to get into the real costs of solar again. Just look at Nevada Solar One and the PS-10 in Spain, how much they cost and how many MWh/year they actually produce.
Those are 4 of the most recently built power plants, 2 nuclear and 2 solar. We have data, both in cost and output. To pretend that data is somehow invalid and projected costs for future projects are more valid is entirely bogus.
Without arguing one way or another on this exercise, I’d observe that back-of-the-envelope totals can be accidentally misleading, and this comes from observing the action here in California.
1) For some time, the most efficient source of megawatts is actually negawatts, i.e., efficiency, and there is a lot of low-hanging fruit, like painting roofs white or with high-reflective paint.
Also, one cannot ignore load-shifting like PG&E demand-response programs, and later, smarter fridges, air-conditioners, etc for home.
The key issue: assuming there is a fixed total demand, that must be met, no matter the cost, is a poor assumption, as shown by long experience here. If there are clear price signals, people respond.
2) There is no time, any time soon, where 100% of any large area is going to be supplied purely by solar. When you listen to utility executives, they talk about mixes, preferably complementary ones.
A more realistic approach, for example, for CSP is to take, say the current power supply distribution for some area (like Victoria), and see what happens when you ramp CSP up over decades. I.e., think of a spreadsheet that lists your power sources, year-by-year, then runs scenarios of different amounts of solar.
Then you can ask questions like:
a) Can you avoid building another coal plant?
b) Can you actually shut off the least-efficient coal plants? When?
c) If you start having a significant number of battery-electric cars or PHEVs, if there is cheap electricity, you can store it their batteries. Smart PHEVs could even “negotiate” utilities as to whether they want to be charged or supply power back. [People are already working on that.] Wind is especially good for this.
d) If you want to shut off the *last* coal plant, what else do you need to do for baseload?
– wind turbines?
– nuclear?
– geothermal?
– storage for CSP?
If you’re in a place where electricity usage peaks summer afternoons [like here in CA or I assume in Oz], solar is very good for load-following, and lets you turn off your least-efficient peakers or use less coal during the day, or lets you save any big, dispatchable hydro for night-time, if you have any. Solar+hydro is a dandy combination.
It makes relatively little sense to spend much money on CSP *storage* until it is such a big part of the electricity supply that it needs to do baseload as well.
But I’d be much more tempted to first invest in some wind turbines with adequate geographic spread.
The issue of shipping. I can do calculations for various locations but indicatively…
These costs are MUCH HIGHER than what the real cost would be as most of the cost is offloading handling, and these costs reduce with scale and distance. average haulage is not over these long distances. For instance Adelaide to perth is 3.9cents per tonne km
1.3 Million tonnes of Steel to Moree from Port Kembla @ 7.9cents per km tonne -> 733km * $102,700 per km = $75,279,100
http://www.artc.com.au/library/news_140508.pdf
166,309 tonnes of Glass to Moree from Viridian (formerly pilkington) Dandenong @ 7.9cents per km tonne to Morree -> 1247km x 13138 per km = 16.3Million
Concrete -> usually produced within 100km else Brisbane to Moree @ 7.9cents per tonne km 481km -> 481km * $155,714 per km = 75.8 Million
NOTE: It would be ridiculous not to quarry the concrete locally. So I will not include it in my total.
Total cost for materials to theoretical plant at Moree in the order of $100 Million Dollars (The factory would be strategically setup near either the glass or steel manufacturing to reduce one leg. Also I’m sure there is a big glass manufacturer in Brisbane or Sydney to reduce costs further
To put this in perspective 100-200Million worth of freight is a small proportion of the 15 Billion dollar per annum Transport Sector of our economy.
Do we have rough agreement – or can we come to agreement on transportation costs for a CSP build out so we can move on to the next question?
PS Obviously we would build at Morree, Mildura, Whyalla Outside perth etc. This is just indicative that it’s not a substantial issue.
Oops, the other interesting thing about all this is the concrete.
See Deltoid, about using coal plant flue gasses + seawater to yield CaOC3 as cement or aggregate, sequestering CO2 (rather than emitting it).
Note: this is technology in early pilot stage, but I would suggest that when one is doing the CO2 analysis of things (CSP, nuclear) that use very different amounts of concrete, the difference between (emitting CO2 via normal concrete) vs (sequestering it in CaCO3-based concrete) may be relevant.
Onesteel have just cut 300,000 tonnes of steel at Whyalla
http://news.theage.com.au/breaking-news-business/onesteel-restructures-management-team-20090205-7y86.html
Tom Blees – “Look at the Japanese experience to see what CAN be done. $1.4 billion USD/GW. Those are the most recent plants built, 2 of them. Why is it taboo to use that real-world data instead of screwy projections from rip-off private utilities in the USA?”
The USA data like US oil reserves is subject to oversight by financial rules (such as they are) and are therefore more transparent than the Japanese data. Generally because of this the USA data is a reflection of the true cost.
Do you have accurate and transparent figures for the Japanese reactors that you can post here?
Barry,
I need rough agreement on cost of transporting tonnage of steel glass and concrete to solar sites then I can go on if we’re going to get to the bottom of the viability of 100% renewables
Matthew Wright
Beyond Zero Emissions
http://beyondzeroemissions.org/
Tom Blees – Further to the comparable costs from my previous reference is this:
“There are several possible sources of variation which preclude confident comparison of overnight or EPC capital costs – eg whether initial core load of fuel is included. Much more obvious is whether the price is for the nuclear island alone (Nuclear Steam Supply System) or the whole plant including turbines and generators – all the above figures include these. Further differences relate to site works such as cooling towers as well as land and permitting – usually they are all owner’s costs as outlined earlier in this section. Financing costs are additional, adding typically around 30%, and finally there is the question of whether cost figures are in current (or specified year) dollar values or in those of the year in which spending occurs.”
So you would need to check the costs for the recent plants and show that the quoted cost included the same things that the other estimates did. I would be interested to see them.
John@42: Rebuilding our power system will be like rebuilding a boat while at sea. It has to stay afloat as each modification is done. We don’t have the luxury of starting from scratch. But the calculations that Matthew and Barry are doing (and which Tom has done in the book) are still worth doing as a first guide.
The costs along the pathway of feasible incremental change are always different from gross averages.
Some questions for everybody watching this blog in Australia:
Where is the oldest coal fired power station in Australia? How can it be targeted to force closure and replacement? What are the rough costs of replacing THAT plant (with CSP, for example)? Are more coal plants on the drawing boards? If so, then where are they and can local groups be formed to block them? If so then these groups need good figures to make a case for an alternative.
re: #24
Hopefully Tom will post something more knowledgable.
1) Jacques Bouchard is a serious guy in the French nuclear establishment, been at it a long time, heads the Gen IV International Forum (GIF) as his retirement “job”.
2) He reviewed history of French power, CO2, etc, including some experimental designs. “No oil, no gas, no coal … no choice.”
3) He said Gen IV would be clearly necessary, and summarized material from the GIF report referenced by Barry.
4) I asked him afterwards to calibrate the meaning of “2030″, which basically meant commercial rollout, i.e., do R&D all along, build pilot plants, get running experience, iterate on designs, and then expect to be building commercially around 2030.
5) I have no particular competence to say whether such dates are:
a) Overly conservative, as people sometimes do with complex projects in any industry where people have been burnt with overly optimistic schedules. Likewise, given that there are brand-new Gen-III designs around, people naturally want to ship those and get a return on the investment. Some may not be so keen to move to something else.
b) Realistic, allowing for all the friction there is on new technologies, with committee cooperation, people still arguing about various flavors, etc.
MY BOTTOM LINE: Gen-IV is important and recognized as such. The timing is naturally in question. From past experience, one can spend money to accelerate projects, but at some point, you can spend an infinite amount of money and not help.
CSP COST AND WATER CONSUMPTION
I have done the cost of a CSP setup to supply 54,000GWh in Victoria (The electricity usage for Victoria in 2008)
250 Million – 177MW Ausra Mirror Field / Boiler setup
250 Million – Siemens equiv Turbine/Gen set
Victorian Electricity consumption 54,000GWh
**How many Mirror Fields do we need 54,000GWh / 387.63GWh = 140
Ratio Storage Field : Real Time Field = 2:1
Ausra Carrizo Field equivalents devoted to real time daylight operation = 47
Field Size real time daylight operation 8319MW
Ausra Carrizo Field equivalents devoted to storage = 93
field size storage 16638MW
Number of Turbine /Generators required = 47 (25% operation realtime 50% operation drawing down on thermal nitrate salt storage)
Cost of Generators $250,000,000 * 47 = $11.75 Billion Dollars
Cost of salt thermal storage @ $30,000 per MWh = 16638MW * 17 hours * $30,000 = $8.485 Billion
Cost of Solar Fields 140 * $250 Million = $35 Billion
Total Cost $11.75 + $8.45 + $35 = $55.2 Billion to power Victoria
This is demonstrated working technology. This setup is short 5000GWh spread over 6 months of the year – the worst months being June and July where the real time supply from solar is upto 1,250MW short over a short period. This shortfall can be provided by the average output of 4000MW installed nameplate capacity of Wind (4000 * 0.33 capacity factor = 1,320MW). This wind installation would require 1111 x GE 3.6MW turbines or 1333 x Vestas V90s, similar to those located at Woolnorth, Tas.
These would cost $8 billion.
Total System Cost = $55.2 Billion (Solar) + $8 Billion (Wind) = $63.2 Billion. (This Solar/Wind Hybrid system has 11,600GWh spare resource that is discarded)
———————-
(Water for 177MW boiler setup (air cooled) and cleaning Mirror field 70,000Litres per day) * 140 Mirror Fields * 365 = 3,577,000,000 litres
http://www.energy.ca.gov/sitingcases/carrizo/documents/2008-04-02_TRANSCRIPT_MARCH_12_DATA_RESPONSE_WORKSHOP.PDF (Page 98 pdf (93 of scanned doc))
versus Loy Yang A 16,000Gwh 34,000,000,000 litres
http://www.loyyangpower.com.au/lyp-tackles-water-conservation.html
To put this in context Cubby Station in the Murray Darling Basin has 500 Gigalitres (Morree and Mildura share this water resource)
Loy Yang A litres per MWh = 2.125 litres per MWh
Carrizo Plant 0.0662 litres per MWh
Matt, please proceed, acknowledging that whilst building plants at Moree, Mildura, Whyalla, Outside Perth is more convenient logistically , these are, climatically, far less suitable sites than central Australia in terms of peak and average insolation (especially re: winter output drop and the relative probability of runs of consecutive cloudy days), as well as available land (perhaps not even an issue in these places — buy out old degraded farmland on a large scale — but please put a cost on this too).
Another important point — the glass, steel and concrete costs per MW are based on a hypothetical (projected) budget for a linear fresnel plant (which was never built). It would be much better if you could work with figures from plants that have actually been constructed, and ideally, use figures from solar towers + heliostats, such as those constructed recently in Spain.
Hi Barry,
Actually the cost projections are very conservative and based on the current Carrizo plain plant that is being built in California, groundbreaking expected later this year.
It would be nice to have the Spanish figures, however I would not use these plants in Australia. I use Ausra technology because.
1. It was developed in our Universities and a power plant (mirror field) has already been built in Australia, and is now being expanded at Liddel
2. Ausra built a 700MWe per annum factory – 4 of these factories would do (54,000GWh) per annum Victoria in 10 years.
The 700MWe reflector/reciever/components factory took 9 months to build, Dr David Mills Ausra says it will take 3months to build/tool up each subsequent factory
http://www.ausra.com/pdfs/Ausrafactoryfactsheet.pdf
3. They proved their 177MW plant by building a 5MW plant in just 8 months to do a test run of their new factory
http://www.ausra.com/pdfs/KimberlinaOverview-101108.pdf
According to Moree Real Estate land is about $1000 per acre
0.405 hectares to the acre $2469 per hectare so it would cost $86.4 Million.
This does not materially effect the costings I put up above.
I believe I have presented a good amount of background information now and have given enough preliminary proof that Solar Thermal/Wind hybrid, can reliably power our innovative future and seriously mitigate against dangerous climate change.
For all the background that I have please see and listen to the Beyond Zero Emissions podcasts @ http://podcast.beyondzeroemissions.org/
Hi Barry,
Here is the rational behind a 30% Cost reduction that is likely with the economies of scale we are talking about based on my figures above..
Carbon Steel $1000 per tonne
Flat Glass $420 per tonne
Concrete $60 per tonne
1,087,460 tonnes Carbon Steel @ $1000 per tonne = $1,087,460,000
133,461 tonnes Flat Glass @ $420 per tonne = $56,053,620
1581760 tonnes concrete @ $60 per tonne = $94,905,600
1,087,460 finished Steel (made into a product industrial average cost $5000 per tonne $5,437,300,000
So for $35 Billion worth of Solar Mirror Field – The cost of the bulk of materials and production is probably under $6 Billion
Dr David Mills has said that with Economies of scale he can reduce this cost by 50% which seems reasonable based on the Materials cost above.
So adjusting the 62 Billion estimate for economies of scale we get $44 Billion for the above Solar / Wind Hybrid.
Matt
Matt #53: Your land value cost estimates for Moree suggest this is not an issue then, I agree.
The cost projections you us are for a proposed plant in Australia that would have been adjunct to a coal-fired power station, used for pre-heating water (I believe — of was this a different one?). They may well have been accurate, but it is impossible to know until the thing is actually built (same deal for the S-PRISM I might add). The Carrizo Plain plant, at 177 MW installed and roughly 30 MW average delivered power, has a current cost estimate of $US 550 million. So that’s $3.1 M installed, or $18.3 M ($AUD 27.3 M) per megawatt or $27.3 billion per GW delivered. For delivery of 6.3 GW, that’s $172 billion (without thermal storage) for replacement of Victoria’s power system. Economies of scale could reduce this by at least 1/3 to 1/2, depending on the time frame involved in roll out.
Your cost estimates for materiel and transport given so far are really useful — thanks, I appreciate you taking the time to document them. But I maintain that based on capital costs of currently built plants, the true cost has consistently proven much higher than the base materiel cost would indicate.
I believe I have presented a good amount of background information now and have given enough preliminary proof that Solar Thermal/Wind hybrid, can reliably power our innovative future and seriously mitigate against dangerous climate change.
You’ve presented some great information, but most of my 6 questions remain unaddressed or incompletely answered, so for completeness could you provide an extra comment where you succinctly reply to each of these? Then we can move on to the second problem of intermittency and the feasibility and implications of backup storage or backup generation capacity, and the cost factors these entail.
A very interesting post here by Charles Barton on Jacobson’s wind reliability and scale up estimates:
http://thoriumenergy.blogspot.com/2009/02/how-much-would-reliable-wind-system.html
I find it hard to follow your figures..
I disagree with your 30MW average delivered power the figure is 44.5 as Moree and Mildura are both 25% capacity factor sites.
My high estimate for 6.3GW constant as you choose to express 54,000GWh per annum is 62.3Billion with storage.
My low estimate for what you call 6.3GW constant is 44 Billion AUD.
Please explain your extra 110 – 130 Billion that you’ve added to the cost of this project.
You can not just convert US dollars to AUD dollars and up the cost of the project in AU, cause we are not importing anything from the USA or anything pegged in US dollars. because the cost of concrete steel and glass has gone down. The cost of Labour has stayed relatively the same (It didn’t go up as the dollar devalued relative to the USD, the other machinery tooling etc can all be handled domestically SEE GEOFF RUSSELL’s point above #40
Barry,
Please see Post #51 which has the proper costings for a
24715MW Thermal, 8238MWe with 17hours storage Ausra Solar Thermal system covering 35,000ha
here are the main costs again, we can not move on while you are grossly over estimating the cost of this system with your 172Billion estimate. Please explain each of the issues you have with these costings. Note the only component exposed to foreign exchange risk is the turbo gen set — however this is a cost that must be paid for a nuclear plant also and therefore is neutralised for the sake of the discussion. When Nuclear IFR vs CSP is put on the table.
—-CUT——
250 Million – 177MW Ausra Mirror Field / Boiler setup
250 Million – Siemens equiv Turbine/Gen set
Victorian Electricity consumption 54,000GWh
**How many Mirror Fields do we need 54,000GWh / 387.63GWh = 140
Ratio Storage Field : Real Time Field = 2:1
Ausra Carrizo Field equivalents devoted to real time daylight operation = 47
Field Size real time daylight operation 8319MW
Ausra Carrizo Field equivalents devoted to storage = 93
field size storage 16638MW
Number of Turbine /Generators required = 47 (25% operation realtime 50% operation drawing down on thermal nitrate salt storage)
Cost of Generators $250,000,000 * 47 = $11.75 Billion Dollars
Cost of salt thermal storage @ $30,000 per MWh = 16638MW * 17 hours * $30,000 = $8.485 Billion
Cost of Solar Fields 140 * $250 Million = $35 Billion
Total Cost $11.75 + $8.45 + $35 = $55.2 Billion to power Victoria
Add 8Billion dollars wind to ride out 0-1250MW winter demand shortage.
—-CUT——-
Ender @ 48: So you would need to check the costs for the recent plants and show that the quoted cost included the same things that the other estimates did. I would be interested to see them.
Apparently not interested enough to read my book.
John @ 50: MY BOTTOM LINE: Gen-IV is important and recognized as such. The timing is naturally in question. From past experience, one can spend money to accelerate projects, but at some point, you can spend an infinite amount of money and not help.
I get my data on what’s possible from the top experts in the field of IFRs, both from within industry and from the people who developed the IFRs at Argonne National Lab. The 2030 figure and others like it are from people who have no particular desire to see this happen quickly, and who put out la-dee-da timelines as if it doesn’t matter how long it takes. I don’t feel we should wait. With commitment from the Obama administration we could have a full IFR up and running before 2015. I believe Barry noted a report here or on another one of his IFR postings that said the same thing.
I didn’t add anything. I simply used a top down approach based on current costs of CSP installations, rather than the bottom up approach based on materiel and transportation costs that you’ve used.
$US 550 million for 177 MW at 17% capacity (Carrizo).
You assume 25% capacity factor for Moree — this is quite remarkable, as even the best desert sites have so far achieved only around 20-25%, and Moree is not an ideal site — indeed it is more akin to Carrizo, CA.
But let’s take 25% capacity factor. $550M/(177×0.25) = $US 12.4 million/MW. 1 MW will deliver 8760/1000 = 8.76 GWh/year. 54,000/8.76 = 6164 x 12.4 = $US 76.4 billion. You say you cannot just straight convert USD to AUD, perhaps so, but there will additional costs, so perhaps 76.4/0.8 = $95 billion.
With a capacity factor of 0.2 this would rise to $95 to $120 billion. This is before we consider backup (thermal storage and/or alternative power generation capacity), energy growth, summer/winter supply, energy dumping or inefficient storage, grid integration, transmission infrastructure, etc.
Barry,
You just don’t seem to understand that you’re trying to purchase 3x the number of Turbo Generator sets that are required. You do not deliver night time power by devoting a new turbo gen set.. You get the existing gen set that is running .2 or .25 capacity factor and you run the night time steam from the Salt thermal store through that.
There are not additional costs — please name these? This is Steel Glass and Concrete, and very minimal other materials such as copper and aluminium which are available locally here in Australia, and therefore do not incorporate foreign exchange risk.
.
your costs do not reflect the cost of a solar field being built in australia from Glass Concrete and Steel, with a local labour force consisting of people who can build domestic buildings, commercial buildings, roads, rail or other infrastructure.
This is low risk and we can get started right away with the first power delivered 1-2 years after the go ahead.
Matthew
Tom: I make no judgement about the specific schedule, as I just don’t have the experience in this domain. I’ve been so buried in other things I’ve only just ordered your book. I wasn’t advocating doing nothing, or saying this has to take until 2030.
It’s hard for me to even start calibration of schedules. Maybe I’ve missed it here, or maybe it’s in your book, or maybe you can help us by giving a summary timeline with enough detail to help see what’s going on and who has to do what. I can’t really tell much from one or two dates by themselves, and I’m speaking as an experienced engineering manager who just doesn’t know this particular domain, but has been involved in many projects. R2-D2 has some useful context.
When I was at Bell Labs, we sometimes got frustrated with the length of time it took to go from something working well in the labs to something we could scale up, produce reliably, run reliably, and support in large numbers… but there were real reasons. Sometimes the issue was getting buyin from somebody else, including state PUCs. I helped create some mechanisms to accelerate this for some kinds of software projects, but the bottom line was that some things accelerated a lot easier than others. Some things just took a certain minimal amount of time, no matter what we did.
Anyway, give us some more of this schedule stuff (or point me at it if it’s already posted.)
Of course, Hansen likes IFR, and he talks to Chu, which may well help… and if Chu can move more DOE effort from weapons stuff into real energy work, it would be a big help.
John, just to give you a feel for it, GE could build a full-size reactor vessel (360MW, which would be the standard size, built in block of two and then clustered) in about a year. Much of the problem is that the Nuclear Regulatory Commission (NRC) takes so long to certify a design and charges about a billion dollars to do it. They’re badly understaffed and underfunded. If the Obama administration decided to get behind IFRs, they could build one without certification at a national lab, and get it done pronto, then the NRC could certify it much faster since they’d have a working model to look at. The recycling center portion of the IFR would likely take a bit longer, but the hardest part of that is being done already and will be commercial scale this year, the rest being easier steps well-proven at Argonne Lab already.
I can’t show you reports on all this because I get it straight from the horse’s mouths. As one may well imagine, big companies are reticent to put in writing what they’re quite sure they can do, not wanting to have their feet held to the fire. So it’s hard to get this in writing, but as I said before I believe Barry pointed to a report saying an IFR could be built by 2015. My own contacts concur. Sorry I can’t be more forthcoming with names and reports, but one must sometimes be a bit discreet.
Matt, that’s a fair point about the turbo generators and thermal storage, and would reduce the single line top down cost estimate I gave — as would economies of scale.
So given current $$ for Carrizo, accounting for fewer turbines and say 1/3 cost reduction by 2020 and a currency conversion rate of 0.9 (to be generous, assuming most costs are embedded within Australia), and adding your thermal storage costs, that would put the figure at $60 to $80 billion, to replace Victoria’s 2008 electricity supply, or roughly $240 – 320 billion for Australia. If we agree on these approximate numbers (it’s probably as close as one can get when dealing with such huge extrapolations), then please do proceed to the next step.
On a side note, you make a valuable point — let’s indeed go ahead, ideally with Govt support, and build a decent sized CSP plant in Australia, to prove up these numbers and the general feasibility of it. But of course a similar argument applies for building a demo S-PRISM plant (in the US, ideally) — I trust you’ll agree.
re: #64 Tom
[I’e lost track of the report Barry pointed to in all this. Where is that?]
Let me try again, because I must not be communicating.
I’m not looking for confidential sources, names, etc. I’m not even looking for PERT Charts or Gantt charts, although a simple list equivalent to the latter, with say 5-10 activities and durations. I’m just saying that for people who are used to doing those things, when looking at an unaccustomed area, it really, really helps to see a timeline in a little more detail.
I think besides lack of transparency, there’s also a need for more research before basing decisions on the Japanese experience. They had to go nuclear early and fast, like France. They’re still working out scenarios that one hopes won’t be needed: http://dx.doi.org/10.1016/j.anucene.2008.12.003
The US regulatory process is difficult and slow because human nature is what it is. We haven’t, as far as I know, had anything close to the Tokai criticality accident. http://www.wise-uranium.org/eftokc.html The executives got wrist slaps, and the company went out of business.
You haven’t had anything close unless you count runaway fuel energy release accidents in general. This is a very incomplete list, but should make the point that commonplace fuels that don’t need to be critically assembled are, due to that flexibility, much readier to kill.
Boron, not needing to be critically assembled, nonetheless has fission-style docility. Maybe I’ve mentioned that before.
(How fire can be domesticated)
Hank @ 67: I think besides lack of transparency, there’s also a need for more research before basing decisions on the Japanese experience. They had to go nuclear early and fast, like France.
Yeah, you just can’t trust those French and Japanese. They’re really losing money hand over fist on nuclear power, but they just won’t admit it. Of course, GE is also lying about it because they have made it very clear how much those systems cost. Everybody who builds nuclear power plants and says they make sense economically is obviously in cahoots with each other and lying. Okay, got it. Thanks for the tip.
This is such a tired refrain. Hank isn’t the first and unfortunately not the last who will trot it out when the facts don’t support their arguments.
The US regulatory process is difficult and slow because human nature is what it is.
No, it’s difficult and slow because the NRC is underfunded and understaffed, because there’s no commitment from the US government to expedite the deployment of nuclear power.
And what, pray tell, does the Tokai accident have to do with IFRs? The wet chemical process they use has absolutely nothing to do with the non-aqueous pyroprocessing system used in IFRs. That accident was a result of two untrained and clueless employees handling radioactive material. It’s like saying wind power is too dangerous to use because some guys fell off a windmill somewhere.
Barry,
I do not know the cost of the IFR plant, and have other resservations, before I can go on answering your last point I will need these cleared up.
I need to know how much it costs to power Victoria with IFR.
Matt:
GEH says $US 1.3 billion per GW for S-PRISM, so let’s be conservative and say $2 billion.
Nuclear plants run at 92% capacity factor and IFRs are likely to be higher because of less need for refueling. But let’s be conservative and say 85%.
Victoria needs 6.1 GW average power delivered, so let’s be conservative and say 7 GW.
Like the CSP plants, most of the construction material for the IFR plants could be produced here, but let’s say it, along with all personnel, is bought in. So use an exchange rate of 0.7.
(2 x 7)/(0.85 x 0.7) = 23.5, i.e. roughly $24 billion (c.f. optimistic est. of $13 B).
The above is subject to all sorts of complications — just as is any attempt to put a real cost on CSP. But it’s a decent rough cut.
Nuclear plants run at 92% capacity factor and IFRs are likely to be higher because of less need for refueling. But let’s be conservative and say 85%.
Just a point about PRISMs: Because they run at atmospheric pressure, the refueling system can be immersed in the primary sodium pool, off to the side from the core. The reactor only has to be shut down for a short time, a few hours, while the old assembly is removed and the new one put in. A staging rack and loading arms inside the primary vessel do this, and then help in transferring the assemblies into and out of the vessel. This is a more involved process, and takes more time, but can be done with the reactor at full speed. So 85% is being VERY conservative. But I generally tended toward such conservatism myself when I did such calculations in P4TP, knowing that there’d be naysayers galore.
Where do you get your figures — where is the plant proposal with sworn testimony such as the Carrizo proposal in front of the Californian energy commission.
And the industrial scale up prototype? Show me these/ numbers for these.
Matt
PRISM reactor power plant for $1,335 USD/kW: Prepared Testimony, Kelly Fletcher, Sustainable Energy Advanced Technologies Leader, General Electric Company before the Energy & Water Subcommittee, U.S. Senate Appropriations Committee, Sept 14, 2006
The fact that the cost of construction material may have risen doesn’t really matter since the materials costs for nuclear run only about $35/kW.
…and further to the $US 1,335/KW sworn testimony figure given by Tom above, there are plenty of technical details on S-PRISM costs (construction through to O&M, fuel cycle cost estimates and fuel supply, etc.), here:
http://www.sustainablenuclear.org/PADs/pad0305dubberly.pdf
Tom#74: $35/kW materials! So where does the money go? I heard (somewhere) that the software production cost of Windows 2000 was about $35/copy – which covered programming and manufacture. We know that the rest of the sales price went to making quite a few of the richest people in the world. So how is the rest of the $1335/kW nuke cost split up?
This 35:1335 ratio explains a lot — like the huge range of cost figures people have been producing on this site lately. Sale prices probably don’t reflect production prices at all. Sounds just like the software business to me!
Well, there’s no arguing the fact that the fabrication of the materials is a costly process, and that the pay scale of the people who build nuclear plants/components is (and should be) quite high. What this DOES tell you is that commodities costs have virtually no impact on the cost of nuclear power plants, and that in a country where interest rates are about as close to zero as you’ll ever see (the USA) there should be NO WAY that the cost of building a nuclear plant should exceed the cost that Japan built them for in the late 90s, much less 5-7 times that price. Somebody’s getting gouged.
Hello,
First issue with that nuclear IFR proposal of yours which makes it impossible for me to agree we need to go anywhere near it- is that paper prices 1000MW THERMAL .. not 1000MWe so you are out by 3x in your calculation… We’ll have to work through this before we can go on, though I have other major issues with it (out of date – The proponent doesn’t propose a specific plant project, so he’s effectively written a marketing document).
Matthew
The price on that document I referred you to is for electrical output, not thermal. Did you even bother to read it? As for being out of date, read my last post: materials costs are immaterial and interest rates are at historic lows, lower than when the testimony was presented to congress. And the proposal is quite specific, to build an S-PRISM power plant of a given size.
Tom,
That’s rubbish, you’ve put up a propaganda piece — there is no way you can build that plant with turbo/generators for that price.. Impossible. Unlike the Concentrating Solar Thermal Plant with storage that is properly costed above.
Matt
If interest rates are low, we can go with Concrete Steel and Glass and start building solar thermal plants. They’ll come online much sooner and present us with Safe secure electricity, unlike the dangerous damaging risky proposal you put forward.
Matt, I’m not sure you can say that a testimony to the senate dating to 2006 is “out of date” or a marketing document, and it supports what’s in the Dubberly et al. conference paper (with 5 authors, not 1, and which is the standard mode of peer reviewed publication engineering, rather than the professional journal). Also, you clearly didn’t properly read the Dubberly et al PDF that I linked to, because even in the abstract, first page, it says:
The calculated fuel cycle cost of less than 5 mills/kW-hr when combined with a plant capital cost of less than 1300 $/kWe1-4 assures that the busbar cost will be competitive with other energy generating systems.
That’s $1300/kWe, with 4 further references. Not kWt. i.e. they are talking about electrical output, not thermal. That’s what the senate figure, updated from Dubberly, was referring to as well. Later in the Dubberly document, they say:
“The reference S-PRISM plant is made up of three power blocks, each of which contain two independent 1000 MWt reactor systems. Since each of the three power blocks has a net output of 760 MWe the total rating of a three power block site is 2280 MWe; however, the size of the site could be limited to 760 MWe until the utility decides to add the additional power blocks.”
They give some costs for 1000 MWt in Table III, but of course if you sum these you find they add to $173 million + $57 M design, + O&M and interest etc, i.e. at 3:1 MWt to MWe, that’s about $520 million/GW + O&M + interest.
They’ll come online much sooner and present us with Safe secure electricity, unlike the dangerous damaging risky proposal you put forward
Matt, your ideology and preconceptions on nuclear energy are starting to poke through the paper facade now — and when shown to be wrong, you are resorting to calling inconvenient costs “propaganda”.
Nothing wrong with them, Barry.. My father was a Nuclear Physicist. You have shown though you have a predisposal to nuclear at the expense of everything else. ie your presumption of $110 billion more expensive power plant for the solar thermal case.
Where is the equivalent pricing. It costs $1 Billion just to get the steam sets up for 1GW of plant (Whether that be rankine cycles for Coal Gas or Nuclear. So how can you get a nucler plant with decommissioning and all those other things listed on page 5 for 1.3Billion. The answer is you can’t.
So what I’m asking for is, the proper pricing of building a plant today, based on a real live proposal to build a large plant. Not some theoretical conference paper.
Ausra Carrizo is a real plant, that has mirror’s being manufactured at an already operating automated factory in Nevada. They have brought a quick 5MW online with the first production from their automated factory, and they’ll be ground breaking this year and completing construction early next year. Then they’ll be pumping power in to the grid.
The timelines in this paper (written in 2003) are for 17 year head way until they get going. Discount rates perhaps? This is the kind of accounting methodology that got us into this mess. So all I ask for is the proposal that is before a (soverign) state planning tribunal to build a plant. Which is what I based all the CSP figures on.
Regards,
Matthew
So Matt, if you have no problem with building IFRs in principle, why use the term “dangerous damaging risky proposal” and earlier remarks such as “[re: ways to boil water] I wouldn’t choose the most complicated especially when that choice increases the likelihood of cancer, serious injury or death, amongst other external costs.?
Re: $110 billion for the CSP — as I explained, this was based on a top-down approach and exchange conversion. You correctly pointed out that this implicitly overestimates the turbine costs, and for the sake of argument, I also reduced the exchange conversion such that almost all costs came out of Oz only. So the calculation was adjusted to a generous $60 to $80 billion — at the extremely generous end of what current data indicates. But it was always simply a transparent approach to the available cost data on the Carrizo plant you continue to cite — as you well know, and so I don’t know why you are implying otherwise.
You’ll also note that my cost estimate for IFR powering Victoria includes the following:
$2 billion per GW, not $1.3 billion
(so there’s your extra turbine cost covered, if indeed you are correct about this and the engineers at General Electric have somehow fluffed it — after all, it was only a ‘theoretical paper’ [a side note: it’s strange how when you talk about future climate change you are not so opposed to considering theoretical work]).
Also, a 0.7 AUD-US exchange rate penalty, an incredibly conservative estimate for the capacity factor (0.85 instead of the more likely 0.95), and an overbuild in the amount of GW delivered (7 instead of 6).
At yet, even with all of those budget penalties, the IFR cost estimate of $24 billion still comes in at 1/2 of your lowest cost estimate for CSP, or about 1/3 of my lower estimate based on the top-down approach. A more reasonable estimate might $14 billion, which brings it to 1/4 or less of CSP, but I’m happy to stick with $24b. And that is not even dealing with the intermittency problem with CSP that only becomes important when it occupies a decent fraction of the total grid supply, rather than a few percent. And yet, we apparently won’t get this is problem, since you refuse to answer the other questions I raised earlier about CSP.
So I have to wonder how genuine your desire to reach zero carbon emissions really is, if it conflicts with what you consider the right and appropriate (not “dangerous damaging risky”) course of action. And in further musing this problem, I have to wonder what your zero emissions plan is for the world, which is ultimately all that matters — CSP and wind for everyone, perhaps?
And finally, I underscore a point Tom made earlier. Neither he nor I are arguing that we should not build CSP (and wind, etc). Indeed, as you’ll see from my recent post on BNC, I consistently argue that we should put in place the infrastructure to help expand CSP, such as a better transmission line connection [and this, and support for other renewables, is ALWAYS included in my public talks]. But you do seem to be saying that we must explicitly EXCLUDE nuclear power. Hence all your energy cards are not on the table, and as a corollary, I maintain that you are not honest about fully solving climate change.
I know this is talking about real nuclear plants and not conference paper theoretical ones, but listen to this guy, you can see he has a lot to say on the issue.
Dan Kammen with the Obama campaign says any plan to increase nuclear energy gets really expensive really fast. Kammon isn’t just a policy advisor, he’s a professor of nuclear engineering at UC Berkeley.
Dan Kammen: “Even if you decide that we know how to manage the nuclear cycle well enough, so that risks are small and acceptable, nuclear plants are tremendously expensive. The nuclear plants that are proposed to come on-line now, new generation of construction, some of these plants are costing more than ten billion dollars each.”
Kammen says the price tag is about a trillion dollars just to replace all the old nuclear plants.
http://news.opb.org/article/1996-candidates-take-global-warming-oregon-campaign-heats/
I think Matt W has shown that it is feasible to generate the baseload power from solar without needing to resort to a nuclear solution. If nuclear was the _only_ way to give us our emission-free power, then it might be worth considering. However the solar options are on the table, so nuclear need not be.
My gut feel is that any sort of nuclear option is bound to have much greater potential for cost and schedule overruns than solar because of extra rigor that would be needed in risk management (to say nothing of the political fallout).
Matt says: not conference paper theoretical ones
So Carrizo is already built and delivering power is it?
Overall, I find it strange and somewhat disturbing that you are willing to accept ‘theoretical’ costs presented by Ausra to a senate estimates committee on the one hand (which I’ve show results in power delivered at 3+ times the cost of nuclear) and yet reject ‘theoretical’ cost estimates presented by the huge multi-national company General Electric – Hitachi to a senate estimates committee. That’s being rather selective with evidence, and reminds me of others in the climate/energy nexus who use the same sort of methods, albeit to argue a different position.
Dan Kammen can say what he likes, but (i) there is no evidence provided to support his statements, Iii) his estimates are not supported by current built costs for Gen III, and (iii) it’s context in a broken regulatory system has already been explained by Tom, repeatedly.
Barry,
5MW Electrical 25MW Thermal
Used the first Mirrors from the 700MW factory that was setup in 9 months and subsequent factories will take 3 months to tool
http://www.veoh.com/collection/NewGreen/watch/v16137710exRtZ7p8
The Kimberlina plant was built in 8 months — Even the manhattan project couldn’t get reactor’s up and running that fast.
http://www.ausra.com/pdfs/KimberlinaOverview-101108.pdf
Where is the Multi Megawatt commercial pilot of the IFR that has been built with the tooling/machining workshops for the IFR? Well there aren’t any tooling/machining/manufacturing workshops
It doesn’t exist does it?
Ausra’s costs are not theoretical, they are real, because they are a real company with a real business plan, and they just opened a real power plant which is pumping electricity in the grid. Where is the light bulb that is being powered by the theoretical IFR plant you propose?’
All I can say is, show me the light bulb, Where is the kilowatt hour of energy delivered?
Dan’s a good guy, I will be chasing him for a radio interview, so he is accessible to the masses.
Here is the proof of the existence of a new 2008 power plant from Ausra
Governator at the opening with the CEO of Ausra
http://www.ausra.com/img/kimberlina0595l.jpg
A line from the Power plant doing a roll showing you the light focusing in
Please provide a video of the 2008 /2009 IFR plant in operation?
Matt
I’m struggling to follow your logic Matt, or to understand your increasingly strident attitude towards nuclear power, or your apparent view that there is no need to continue to roll out new and more advanced energy solutions.
– Fast spectrum reactors exist and are powering light bulbs, as has been stated in the above post. An IFR is a sodium-cooled fast spectrum reactor with an on-site pyroprocessing facility.
– General Electric and Hitachi are real companies, rather larger than Ausra, and are responsible for pumping vastly more electricity into the grid
– Following your logic, we cannot ever use 20 MW wind turbines because none are powering light bulbs.
– You haven’t explained why so-called ‘theoretical’ plant proposals are unacceptable to you, and yet ‘theoretical’ models of future climate change are. But since you follow that logic, the Carrizo 177 MW plant is unacceptable, since it is only ‘theoretical’ that a solar thermal plant could ever be that large (following your logic to its reductio ad absurdum)
You still haven’t addressed my 6 initial questions on CSP.
Oh, and here is your video: http://www.youtube.com/watch?v=mJj-SvNjqEI
No it is not theoretical, they have purchased the land and the mirror’s are coming off the production line, and are being warehoused, They will shortly be moved to the laydown site next to the mirror field construction zone. Where is the 1GW or 360MW or whatever reactor being built as we exchange posts? It isn’t.
Try me again with the reactor that has been built in the last couple of years using the exact plant, tooling, etc of the industrial scale model. Industrial engineering is a lot more work than just taking something out of the lab and building it. I assume you haven’t worked in industry before, to have overlooked this important fact.
I never wanted to use 20MW wind turbines, 2.5-3.6MW units that are currently in production in the 1000s (3MW,3.6MW) are fine by me. No need for 20MW. We have more than adequate resource to substantially power Australia with the available wind resource on cleared agricultural land using 3MW turbines.
I will answer the question of theoretical climate science vs theoretical nuclear conference paper a little later when I have some time.
Industrial engineering is a lot more work than just taking something out of the lab and building it. I assume you haven’t worked in industry before, to have overlooked this important fact.
Now you are just being cute — or perhaps deliberately insulting? Not sure. I’ll leave others to judge. But I’ve personally got no more time for your silly games.
Matt @ 86: Dan Kammen with the Obama campaign says any plan to increase nuclear energy gets really expensive really fast.
Don’t get me started on Dan Kammen. He and I went around during the Obama campaign. He knows better than what he’ll say, being a big proponent of renewables. His cost figures for nuclear were so ridiculous that I sent him several more realistic appraisals, helpfully suggesting that maybe with all the work of the campaign he hadn’t had time to see them. He wrote back telling me he was well aware of them, but then arguing that if that’s what the utility companies say it’ll cost then that’s what it costs. He even did a “study” on the costs of nuclear power that he was good enough to send me. The upshot of it was that his study consisted of a litany of the financial horrors of the nuclear power debacles of a couple decades ago, concluding that we can’t assume things will be any better next time around so we’d better assume the worst case scenario. Ergo, nuclear is too expensive to contemplate. The unintended irony is that he had a graph of the projected costs of various nuclear power plants at the end of his paper, showing the AP-1000 coming in at about a tenth what he talks about with reporters, giving the lie to his whole position with his own data. Fortunately he didn’t end up as secretary of energy.
Matt @ 89: The Kimberlina plant was built in 8 months — Even the manhattan project couldn’t get reactor’s up and running that fast.
Ausra doesn’t answer their phone so I have to go by what they estimated for a capacity factor themselves: 20%. That means this 5MW “power plant” is producing the equivalent of a 1 MW nuclear plant. And it only took 8 months to build and cost $15 million USD, for an effective capital cost of $15 million/MW. The first of a kind ABWRs built in Japan have a 1315MW rating. At the average capacity factor for nuclear power plants (which the PRISM will improve on, since it only takes a few hours to refuel), that would produce an output of 1183MW average. They built it in 51 months. At the rate the Kimberlina plant was built (1MW/8 months) it would take almost 800 years to match the capacity of one ABWR.
It’s thermal steam 25MW Electrical 5MW — And they do answer there phone I just rang them to check.
This plant only has a mirror for daytime operation, and yes it is in a 20% resource area — In Australia we would build them in 25% Annual sunlight resource area.
It is not equivalent to a 1MW Nuclear plant annual output it’s equivalent to 1.5MW of nuclear plant annual output. Because a nuclear plant is designed to deliver power when you do not need it early in the morning and might as well be blowing steam at this time.
All the details were in the data sheet I pasted the URL for — it would have helped if you had read the datasheet. It was built to test the Mirrors, Factory, tooling, installation prior to sending all the equipment for lay down at Carrizo plant after mid year.
http://www.ausra.com/pdfs/KimberlinaOverview-101108.pdf
The beauty of installing Mirror lines, is that you just increase your Labour force, there are no blockages. The 177MW module will take 1 year to build from laydown of equipment onsite Ausra’s first factory can manufacture 700MW electrical of mirrors per annum, and these factories can be brought online in 3 months from lease of a factory floor area.
Your 800 year estimate is ridiculous, they had to do site remediation / clean up because this site was used for another purpose and is close to population centres. It is not an ideal solar thermal site, just somewhere they could test all their equipment/procedures / labour contractors and be able to show bankers/ investors and government regulators.
In 10 years we can easily install enough power to predominately power Australia, the USA etc. At heightened prioritised speed we could do it in 5 years and at military Manhattan project speed we could do it in 3 years.
This is an interesting story about how purveyors of poison put out super optimistic nuclear power plant costs
A Contract to build 1600 MWe EPR reactor at Olkiluoto in 2003 is still not delivered and unlikely to be delivered by 2012. The project was supposed to cost 3.0 Billion Euro’s or $5.9 Billion dollars AUD but is 70% over budget so is now at least a $10 Billion AUD project.
So to meet Victoria’s day and night power needs with pure nuclear you’d need 10GW or 7 Olkiluoto size plants for a total cost of $70 Billion dollars. The only problem is that the plant will take at least 10 years to deliver. So you have waited for the complete loss of arctic summer ice before you even see power delivered.
Where as by installing 50% of your solar thermal on year 5 you are marketing 2 billion per year of power, rising to 4 billion a year by year 9. And still not one unit of energy has been delivered by a nuclear plant.
So to go nuclear shows a belief in a zero carbon future at some other time while Victoria goes through desertification and the arctic ice cover depletes , ie well in the future. But going Concentrating Solar Thermal is about breaking ground now, diverting glass steel and concrete along with molten salt and standard power blocks now and getting power online, and coal offline in a matter of a couple of years, growing to 100% within 10 years.
http://www.world-nuclear-news.org/newsarticle.aspx?id=24553
Huge potential costs for OL3 delay
29 January 2009
Billions of euros are involved in the arbitration between TVO and the Areva-Siemens consortium… [Ed: snipped the rest — people can click on the link you have provided to read the rest — this is a place for your own comments, not for regurgitating huge slabs of text from other websites]
This thread has been dominated by costs and while some of the discussion
has been useful, there’s a few too many one-shot inductions going on. e.g.
this solar|nuclear plant cost $x therefore this is THE solar|nuclear
cost. This is all a bit silly.
A crucial component of Tom’s P4TP was that IFRs should be a commodity.
Likewise solar of the CSP+storage variety should be a commodity. You
only get commodity level savings when you have clear agreed standards. Most
companies hate standards and fight them tooth and nail. Put in clear
mandatory design and interface standards and prices on both technologies
will drop. Companies will try and complicate the standards to
give their pup any edge they can.
All the details were in the data sheet I pasted the URL for — it would have helped if you had read the datasheet.
Matt, a little rich, given your misreading (or lack of reading) of the S-PRISM paper.
I hope the full 177 MW Carrizo plant can be done in 1 year, and that economies of scale bring down the cost, and that methods for thermal storage continue to be developed, and that appropriate transmission infrastructure is supported by government to allow proper development of solar, wind and wave resources. Indeed, I earnestly hope that CSP and wind energy continue to expand greatly worldwide. So does Tom — if you bothered to read his book, you’d know this.
Our point of difference is this — we see a need for a diverse energy mix to maximise our chances of a successful energy supply and climate change mitigation outcome — with the level playing field conditions to allow the most efficient, most economical and most reliable sources to play their maximum role. You, on the other hand, demand that some options (e.g. nuclear power) be explicitly excluded from contention or support, with phrases like your most recent “purveyors of poison” thrown in for a bit of dramatic flair.
Such behaviour frankly makes your whole position/plan, even the many creditable parts that I support, smack of a disingenuous ulterior motive. So I’ve got no further time for you (not that this will bother you, I’m sure). I’d rather discuss these issues rationally, with the many rational folks out there who can appreciate the wider picture. Not waste any more time on this back-and-forth game of charades.
Note: The original IFR critique, to which this post responds, appears to have been yanked off the FoE website. No matter — all of the statements in green in the above post cover what was said.
A graph speaks a thousand words…
http://nucleargreen.blogspot.com/2009/03/wind-electrical-generation-data.html
FoE critique is now here:
http://www.foe.org.au/anti-nuclear/issues/nfc/power/ifr
Still no link back provided to this post — I wonder why.
Note the improvement I suggested in comment 4 to my no-reactor-has-built-a-reactor remark. Very true, but not quite what I meant.
(How fire can be domesticated)
[Ed: Whoops, fixed]
Thanks for the info found it very useful
hi, i’ve only today (April 1) seen this webpage hence lack of response to substantive comments and to Barry’s complaint about the lack of cross-links from the FoE website (which has hopefully now been rectified to Barry’s satisfaction). Debate on IFR is welcome but difficult since this BNC webpage is a critique of an earlier version of the FoE webpage and vice versa. Anyway I trawled through this BNC webpage hoping to find something to allay concerns about WMD proliferation risks but found nothing reassuring and nothing that hasn’t already been addressed in the updated (March 12) version of the FoE webpage. If anyone wants to critique the WMC section of the updated FoE webpage, that would be welcome.
http://www.foe.org.au/anti-nuclear/issues/nfc/power/ifr
This is exactly the same as the AGW deniers saying “its cold today therefore AGW is wrong”
You can’t post data from one wind farm and draw any conclusions at all. You could do exactly the same for a nuclear power station when it is being refuelled once a year.
And before you say that refuelling can be planned so can lulls in the wind. Weather forecasting is quite advanced and this lull could well have been predicted and allowed for exactly like a nuclear power station refuelling operation.
May as well post this reply at Charles’s site as well.
Thanks Jim, I’ve updated the above post to reflect this cross-link.
The IFR was designed to (amongst other things), burn up all of the spent LWR fuel, enriched uranium and weapons-grade plutonium stockpiles that have been accumulating for the last 50 years, which most of us would like to get rid of (and in some useful way that produces cheap energy as a byproduct) rather than managing for 10,000 years. When operated under a GNEP type programme, initial manufacture of the fuel rods would only need to take place in those countries which already have reprocessing facilities.
I don’t see how this ‘fails the crucial proliferation test’. The net effect of the IFR will be reduced availability of bomb material worldwide. What is your solution to eliminating the existing stockpiles if it is not via fission transmutation? You have the criticisms, but not the answers.
In theory, IFR is great (part of that theory including Blees’ “rigorous international oversight”). In theory, reprocessing/MOX/breeders were/are great. We don’t live in theory. In practice, reprocessing/MOX/breeders have been disastrous. The intention was to recycle uranium and to get rid of troublesome plutonium and to use it as an energy source in the process. In practice, stockpiles of civil, separated plutonium have increased year after year, decade after decade and now amount to 270+ tonnes – enough for 27,000 bombs. That is disgraceful, firstly because of the scale of the problem and secondly because its resolution could hardly be simpler – suspending or reducing the rate of reprocessing to reverse the trend of ever-increasing stockpiles of separated plutonium. Similar commercial, political and sometimes military imperatives will affect the development of IFR. When you say: “The net effect of the IFR will be reduced availability of bomb material worldwide”, you ought to say ‘could’ rather than ‘will’.
It is on the basis of the following (agreed?) facts that IFR fails the crucial WMD proliferation test. 1. IFR can be misused to irradiate targets/blanket to produce weapon grade Pu. 2. Pu can be separated from IFR fuel using conventional Purex reprocessing. The Pu will be weapon grade if the irradiation was deliberately curtailed or reactor grade if optimised to produce electricity.
As for eliminating global stockpiles, it’s an immensely difficult question, suffice it here to say that FoE has been working hard to address one small part of the problem – doing everything we can to force Australian government agencies to provide information on the stockpiles of Australian-obligated separated plutonium and doing all that we can to persuade the government to adopt a more responsible policy on that front – no Australian government has ever refused permission to separate Australian-obligated plutonium from spent-fuel, even when that results in stockpiling, as in Japan. FoE is also working with academics, medicos, other NGOs etc to persuade the government to enforce tighter safeguards arrangements to Australian-obligated nuclear materials. Years of hard work are starting to pay off in that regard.
Every country that’s developed nuclear weapons has done it separately from, and usually prior to, nuclear power program development. The anti-proliferation aspects of IFRs have been fully articulated. That being said, I don’t disagree that fissile material must be overseen by a responsible international agency, a point to which I devote a great deal of attention in Prescription for the Planet. But the fact remains that if someone wanted plutonium for a bomb they would be far better off creating it at a small research reactor rather than jumping through the almost impossible hoops it would take to make it at an IFR site. Why choose the hands-down hardest way when there are far easier ways? It would require the connivance of everybody at the plant to get the raw material out of there, then an expensive and highly visible PUREX plant to separate it. With all the spent fuel sitting at LWRs and all the research reactors scattered around the world, the idea that IFRs would be used to surreptitiously produce plutonium for a weapon is so ludicrous.
Fabricating such improbable scenarios does nothing to address proliferation. Nuclear power is not going away, like it or not. Wouldn’t it be far more effective to work for an international regime and the safest, most proliferation-resistant design of nuclear power plant to be standardized and deployed under comprehensive and dependable supervision? The false dichotomy of nuclear or no nuclear only exacerbates the threat of proliferation, for it hampers the sort of international cooperation necessary to address that very real issue. China, India, both Koreas, Russia, France, the USA, Britain, Israel, South Africa, and Japan (did I forget anybody?) are all going to build more and more nuclear plants. A number of other countries will join them in the near future. Do we engage with the rest of the world and do our utmost to see that it’s done right, or do we continue to pretend that if we just close our eyes real tight nuclear power will go away?
For the record, I’m not crazy about MOX reprocessing and plutonium separation myself. All the more reason to build IFRs.
Reprocessing, like enrichment, involves a collection of sensitive technologies that – bottom line – must be rigorously controlled as mentioned in this post as well as Tom Blees’ post. It simply must be done and the recent acknowledgement of the IAEA’s role in this effort by the Obama administration, as well as Australia’s recent interest in international non-proliferation efforts, are positive signals of a bright future for nuclear technologies.
It is not rational to expect the world to abandon nuclear technologies which provide not just very low carbon electricity, but also energy security, important medical and industrial isotopes and significant contributions to science.
In this context, one must objectively evaluate and work to minimize overall risk. Is it challenging to manage nuclear proliferation issue? Certainly. Impossible? No. More effort than justifies the benefits mentioned above? I do not believe so.
As Tom says, the link between nuclear power and weapons programmes is very weak if it exists at all. Non-proliferation funds flowing around the world from governments and NGOs alike are not being directed toward nuclear power. They are working to convert many research reactors from the use of highly enriched uranium (HEU) to low enriched fuel, similarly convert the process to produce a critical medical isotope (Tc-99m), and return spent HEU fuel to the country of origin. North Korea has no nuclear power plants and Iran’s first has yet to be fully commissioned.
Efforts to increase the transparency and the heighted safeguards of related activities noted by Green are helpful in this regard. The recent success noted in the post is another sign that Governments are taking nuclear technology’s role seriously and are beginning to put in the effort to ensure its continued safe and secure use for many decades to come.
Unlike Tom Blees, I do support the limited and strictly controlled production / irradiation of MOX fuel in power reactors. It will be some time before the world experiences rapid IFR deployment and I see no reason not to consume some of the Pu stockpile. I am also aware of advanced fuel designs which will combine Thorium and Pu. Such a fuel supports the sustainability of nuclear power as well as the consumption of the world’s Pu stockpile.
I look forward to reading more about Tom’s ideas. His book arrived from Amazon (finally!) just today.
No point re-rebutting Blees’ comments, we’re going around in circles. Except to point out yet again that the manifold links between nuclear power (inc. thorium/U-233) and WMD are discussed in detail at: . See also relevant papers at .
Barry – IFR theory has been comprehensively addressed, you might see some value in initiating a forum on the realpolitik of IFR. Some issues that could be addressed are: 1. Suggestion that IFR be limited to nuclear-weapons-capable states (long history with similar debates).
2. I think someone has suggested state-owned IFR plants (lots to discuss).
3. In theory IFR will reduce global stockpiles of fissile material – but what will happen in reality and how to overcome the formidable array of commercial, political and military interests responsible for, amongst other things, 270+ tonnes of civil separated plutonium from theoretically-attractive reprocessing/MOX/breeders?
4. How do we get from the current flawed and under-resourced safeguards system to “rigorous international oversight” and what happens if we get IFRs without rigorous safeguards? Do IFR advocates have a workplan to do what you can to bring about improvements in safeguards (as FoE does) – if not, that workplan is overdue and would be a good topic to flesh out.
It’s good to see some civilised too’ing and fro’ing with Jim/Barry/Tom/GRLC.
There seems to be some technical agreement with Jim now relying on an “in theory … but in practice” line. I’m interested in who the bad guys might be and what they might want.
Is it terrorists? Or is it rogue states? Terrorists are an annoying bunch but their current track record in death in destruction is outdone by thousands of fold by salmonella. Even if the terrorists had a nuke, I’d bet on salmonella still causing more death and suffering.
Rogue states worry me more. Russia is in all sorts of trouble at the moment with the total collapse in public health following the collapse of the old soviet union and life expectancy in free fall. Internationally puffed up, but domestically a basket case. India? Pakistan? China? I can think of a couple of edge case global scenarios.
Scenario 1: lets pretend we disallow nuclear electricity generation .. like we have this power :). If renewables don’t fully replace current and woefully inadequate power generation, political instability and public health will decline further. Generating friction with a neighbour has a long history of diverting instability at home so India/Pakistan could come to blows. Ditto Russia and … pick a state, any state. None of these possibly waring parties will need to worry about putting a nuclear electricity station to uses it wasn’t intended for. They have a military to do their bidding.
Scenario 2: we pull all stops out to develope IFR. It seems to be agreed that this can give us low-carbon (using a lot of concrete) power in large amounts in a few decades (Jim?) with some risks seen as show-stoppers by Jim and negligible by Tom/GRLC.
The current plummetting health of people in Russia together with the always dismal health of at least a couple of billion other people seems to be locked in by scenario one. Even if a few luck countries can get away with solar, wind, geothermal, does anybody (Jim) seriously suggest that the other 3 billion can industrialise with these energy sources and that these sources can save Russia? My judgment tells me that the risks associated with scenario 2 are worth taking to avoid the near certainties of scenario 1.
hi Geoff
Glad that there is finally agreement on the WMD potential of IFR. Disappointing that that WMD potential wasn’t spelt out earlier on in the discussion. Also disappointing that there seems to be no acknowledgement from IFR advocates that promoting dual-use technology carries with it the responsibility of engaging in the laborious work of trying to force improvements in safeguards – my post on that topic remains unanswered (above in this webpage).
My main concern is states (rather than terrorists) tapping the WMD potential of IFR. The reason for that is that it is not straight-forward building nuclear weapons even once the most difficult step – accessing fissile material – has been accomplished. Sub-state groups might be gifted or buy or steal weapons but generally couldn’t manufacture them.
Re waring parties
– some states could build weapons in months thanks largely to their civil programs
– Iraq’s 1991 ‘crash program’, diverting HEU from a research reactor, is one example of a scenario you think unlikely
– it raises the major but little-discussed problem of conventional missile/bomb strikes on nuclear plants during war. Already a history of this in the Middle East. God forbid if there’s war in, for example, north-east Asia anytime soon.
The clean energy ‘deep cuts’ studies referenced at
might be of interest.
I’m gradually adding to the FoE IFR webpage
.
I wouldn’t say there is agreement, but the need for oversight and safeguards is acknowledged. Tom/GC/Barry don’t agree with you on the level of WMD risk that IFR poses, but everybody I think agrees that nobody needs safeguards on PV cell manufacture.
But the thing that has swung me from a lifelong anti-nuclear stance is the pressing need not just to replace an existing energy infrastructure but to ensure that any additional energy infrastructure isn’t coal. “pressing”, of course, doesn’t really capture the urgency. Have a read of Laurie Garrett’s “Betrayal of Trust” to see what has happened in the USSR since 1991. The veneer of civilisation is thin and easily ruptured. A global shift in rainfall patterns and an increase in meat production in developing countries has already added 100 million people to the undernourshed count. Plentiful energy can’t fix all such problems, but its a good start.
Its particularly depressing to see Rudd planning to spend $43b on giving everybody movies on demand instead of phasing out coal.
Jim, Tom and I agree completely that a clear plan for enforcement of safeguards is required. Some authors have suggested that GNEP, if widely adopted, will serve that role. Perhaps, but Tom certainly sees something rather bolder and more comprehensive.
I’d urge you to read chapters 10 and 11 of Prescription for the Planet, pg 263-317, where Tom maps out his vision in considerable detail. I’ll be reviewing these crucial chapters soon in part V of my multi-part review of this book.
Also, for some reason your links are not inserting — you can use “a href” style or simply plonk them in with the http in the front.
No Solar Thermal plant can be used for any sort of weapons proliferation. And Employee insurance premiums would be much lower at Solar Thermal plants.
All about Solar Thermal power plants that will use just 2% of our annual sales of concrete steel and glass to construct over 10 years. Why would you do anything less.
http://beyondzeroemissions.org/Technology-Review-Solar-Thermal-Power-Towers
Why would you do anything else? Here’s why:
http://bravenewclimate.com/2009/03/18/the-solar-fraud/
http://bravenewclimate.com/2008/12/21/renewable-energy-cannot-sustain-an-energy-intensive-society/
http://bravenewclimate.com/2009/01/06/prescription-for-the-planet-part-i/
http://bravenewclimate.com/2009/01/16/put-all-energy-cards-on-the-table-to-fix-climate-change-fully/
http://bravenewclimate.com/2009/02/12/integral-fast-reactors-for-the-masses/
http://www.world-nuclear.org/info/inf122_heavy_manufacturing_of_power_plants.html
—-excerpt follows—-
For very large generation 3+ reactors, production of the pressure vessel requires, or is best undertaken by, forging presses of about 14-15,000 tonnes capacity which accept steel ingots of 500-600 tonnes. These are not common, and individual large presses do not have high throughput – about four pressure vessels per year appears to be common at present, fitted in with other work, though the potential is greater than this. Westinghouse is constrained at present in that the AP1000 pressure vessel closure head and three complex steam generator parts can only be made by JSW. Areva has a little more choice.
Reactor vendors prefer large forgings to be integral, as single products, but it is possible to use split forgings which are welded together. These welds then need checking through the life of the plant.
Westinghouse says that the minimum requirement for making the largest AP1000 components is a 15,000 tonne press taking 350 tonne ingots.
The very heavy forging capacity in operation today is in Japan (Japan Steel Works), China (China First Heavy Industries) and Russia (OMX Izhora). New capacity is being built by JSW and in South Korea (Doosan), France (Le Creusot) and is planned in UK (Sheffield Forgemesters) and India (Larsen & Toubro). In China the Harbin Boiler Works and Shanghai Electric Group are bidding for AP1000 work which will require very heavy forging, so they can be expected to install that capacity rapidly if required. Nothing in North America currently approaches these enterprises.
However, another development is Westinghouse going upstream and setting up factories in USA and China to produce modules for AP1000 reactors. ….
Barry, Jim Green’s article has been updated at the end to highlight you stating that “To produce weapons-grade plutonium you would have to build an IFR+HSHVHSORF (highly specialised, highly visible, heavily shielded off-site reprocessing facility)”. Jim Green seems to be taking this as you admitting that IFRs can produce weapons-grade plutonium. But it seems that off-site processing would not be part of an Integral Fast Reactor as it would fail to be Integral.
Is it possible to elaborate on how these facilities are highly visible? I remember you saying in a talk that remote sensing could be used to discern PUREX processing from pyro-processing, meaning that nations could no longer hide weapons grade material production behind a nuclear power program.
For me it could help to clarify the position that IFRs cannot produce weapons-grade plutonium. Sorry if this has already been addressed.
Hi, interesting discussion Barry.
2. What do you do when you have a few cloudy days in a row, especially in winter when generating capacity is at half the summer insolation? How much extra thermal storage do you build in to your system to stop extended blackouts?
I have always wondered about this exact question, and wondered whether the heat could also be backed up by a few large onsite bio-gas tanks? You know, methane biodigesters (like Kompogas in Europe) or Biochar gas, stored in tanks for just this occasion? Not sure how large the tanks would have to be to top up the heat for a week of cloud.
Would it be economical to have huge sheds of good old fashioned WOOD pellets just for these occasions, and have a backup furnace that can pipe some extra heat to the turbines when required? When we are dealing with enormous industrial areas of this magnitude a few huge farm sheds of wood lying there aren’t really going to add too significantly to the land required, especially given the importance of electricity to the local economy.
Hi Ender,
Also not all the CSP plants would have to have storage as the peaks when it is needed would be quite sufficient for perhaps 30% of the CSP plants as we do not need as much supply at night.
nice point there regarding off-peak demand, although don’t forget night time demand may rise a bit when the “Better Place” electric cars start pluggin in and charging overnight.
Also, found this, which makes me wonder how much governments are subsidising the insurance costs and whether or not there is far more to “insurance” in the real world of nuclear accidents, however unlikely, than implied by even by your quote?
One of the biggest of several hidden subsidies for nuclear power is that it is only required to pay a small fraction of the cost of insuring fully against claims from a Chernobyl-style disaster, or worse:
“… in the United States, the Price-Anderson Act limits the nuclear industry’s liability in the event of a catastrophic accident to $9.1 billion, which is less than 2% of the $600 billion guaranteed by the Congress. In any case, $600 billion is considered to be a gross underestimate …” (Helen Caldicott, p. 32). There are similar limitations on liabilities in other countries around the world, including the UK.
Full insurance against nuclear disasters would completely demolish any economic case for nuclear power.
Other hidden subsidies include:
* The costs of providing protection against terrorist attack for nuclear plants, and for trains and ships carrying nuclear fuel and nuclear waste;
* The costs to us all arising from the fact that any such protection can only ever be partial;
* The cost of decommissioning nuclear plants. An estimate in 2006 by the UK Treasury for the cost of decommissioning the UK’s old nuclear power stations was £90 billion;
* The costs born by national governments in that ultimately they must underwrite all risks, as evidenced by the way the UK government had to bale out British Energy in 2005 at a cost of £5 billion;
* The costs arising from nuclear waste that will be dangerous for thousands of years. These costs will be born by future generations but they will receive no compensating benefit.
http://www.mng.org.uk/gh/nn.htm
Remind me who insures against a large hydro dam breaking? Or a hostile nation state invading? Or a disease pandemic? etc. etc.
Yes, but that’s not the point is is? It has to be included in the overall ‘price to society’ of the energy source or we’re cooking the books.
Hey, if nuclear works out as safe and cheap as you’re saying, then why not? I’m just waiting on more data. Maybe new nuclear technology will come out so cheap that the overall cost to society drops, including insurance.
But it’s a significant economic factor that solar thermal doesn’t have to deal with. If a terrorist blows up a solar thermal plant, oh dear, we have to rebuild some glass and concrete. But if a terrorist DOES manage to blow up a nuke with high level radioactive waste going everywhere, we have to evacuate a whole region.
What is the economic cost to ‘lose’ Melbourne of Sydney in this manner, even if everyone is evacuated in time?
Seems to me a risk management exercise. If the chance of something, although incredibly small, is still incredibly NASTY, we HAVE to insure against that chance.
How much is this insurance really going to cost? DO insurance companies really insure against such scenarios? 😉 Or is “moving Melbourne” just so unthinkable a scenario there’s no policy in the world that will cover it?
I know this is all alarmist hippie crap to some reading here, but CAN you 100% guarantee this will not happen? With solar thermal & a mixed renewable grid, we can.
CAN you 100% guarantee this will not happen? With solar thermal & a mixed renewable grid, we can.
Can you 100% guarantee that solar thermal and a mixed renewable grid will keep the lights on? Germany’s experience has shown that to be a pipe dream (please, Ender, don’t bother to quibble, I’ve heard all your arguments and I simply don’t buy them. I know how to count.). In which case you are left with the other options: fossil fuels or nuclear. With the risk assessment studies of the PRISM indicating it’s about as meltdown proof as can possibly be expected, I’d opt for IFRs any day of the week.
I can’t, but it seems others more specialised in this stuff can.
Dr Mark Diesendorf
http://www.cana.net.au/documents/Diesendorf_TheBaseLoadFallacy_FS16.pdf
It’s not about ‘baseload’ and the theoretical capacity to use a combination of wind and natural gas to simulate a coal-fired power station. It’s about managing variability, storage, backup and redundancy (overbuild) across a country-scale renewable grid.
Don’t we have to get a move on though? 350 and all that? Clock is ticking before the big tipping points kick in and nature takes over?
I don’t get this faith in Gen4 when the reactors are so far away. If these super-safe, super-cheap Gen4 reactors are not built till about 2030 (according to wikipedia, whatever that’s worth)…
http://en.wikipedia.org/wiki/Generation_IV_reactor
Any time I hear “oh, but they’re coming in about 20 years” I think of the abundant fusion energy we were all supposed to have by now.
By 2030 we’ll already have 2 decades of experience with renewables! Wind won’t be on his own, but will have heavy hitting baseload friends such as solar thermal, CETO wavepower (+ desal at night!) and geothermal.
Next year we should get to test a whole town running on solar thermal. (Although only Cloncurry, QLD, a small town of 2,340 people it should give us a good idea for that region at least).
http://www.theaustralian.news.com.au/story/0,25197,22700605-2702,00.html
http://en.wikipedia.org/wiki/Cloncurry_solar_power_station
How can you make such bold assertions about the costs of cheap *modular* Gen4 technology when we can’t walk up and kick one yet?
I don’t get this faith in Gen4 when the reactors are so far away. If these super-safe, super-cheap Gen4 reactors are not built till about 2030 (according to wikipedia, whatever that’s worth)…
It’s not worth anything in this instance. It looks like we’ll be building one of these things very soon, perhaps even starting by the end of this year. Lots of serious groundwork is being laid that you won’t find mentioned in Wikipedia or anywhere else.
Care to share, Tom?
or anywhere else.
Then there’s no way I can make a decision.
I’m hoping these things can be as *cheap* as you guys say, simply because we need all the options we can get.
Even if the actual energy infrastructure costs come in cheaper than renewables with backup, it would probably be the “externalities” that blow it out. Not including the real cost of extra security and insurance issues would be just the same as not including the real economic impacts of global warming in the coal budget. Society pays, but the books are cooked (a bit like the planet).
Again, if a terrorist blows up a solar plant, it just doesn’t matter.
Care to share, Tom?
I’d love to, but at the moment I can’t. Politics, especially nuclear politics, can’t always be transparent. I know politicians who are convinced that IFRs are a great idea but they won’t utter such a sentiment in public. Having to pander to the public’s prejudices and irrational fears is an unfortunate corollary of democracy (such as it is). So a lot gets done behind the scenes. There seems to be little alternative.
Thought so. Still, its nice to hear something encouraging on this front.
This is a brilliant post. My commendations to all the authors 🙂
Dir Sir,
Well done on this website and the direction you travel. I suppose I am one of the ‘denier’ crowd, not in the sense that I deny climate change, that seems to be a given, just the warming/cooling fluctuations and how much of that can be attributed to mechanized mankind. That said, I will not be unhappy at the demise of using coal and oil for the lowly purpose of boiling water. They are far more valuable compounds than that in my opinion. The R&D into IFR and LFTR is in my opinion well overdue in Australia, and I commend you for getting the word out into the Blogosphere and other more mainstream media outlets. I quoted you at some length, with appropriate citations, on the Facebook pages of the Greens, Labor and Liberal. Very frustrating as from the Greens and Labor the message is succint, “anything NUCLEAR is EVIL”, and the Libs seem to do an “ummm…ahhh…well…we’d rather not say….really”.
A very hard task, getting to have mainstream politiks to listen to this good sense. Hey, if it doesn’t work, bin the idea. It deserves a lot more than just being ignored.
Best of luck from the ‘other side’ ( a horrible contractor who has had industry exposure to coal, oil, power generation, power transmission and lots of other terrible things 😉
In all, excellent work.
Regards, Mark.