Debate over large-scale energy storage is a regular theme in the comments on this blog. The post is intended to be a place to centralise this discussion. Some questions that might be considered in the comment thread:
1. What is the cost (per Watt hour, kWh, MWh, GWh — how does this cost scale up, and how does this scale as higher levels of reliability are required, e.g. energy delivered on demand 90% vs 99% vs 99.9% of the time)?
2. What is the energy density of the proposed storage technology currently, and what are its physical limits? (i.e., how good can it get, with perfect engineering, and how long can the energy store be held?)
3. If the storage technology becomes cheap, what is to stop baseload plants like coal and nuclear from undercutting renewables, given that they can charge large batteries in low-demand times and then sell the power during peak (high-price) periods?
4. What are the material inputs for the storage system, and how does this effect the energy returned on energy invested of the paired energy technology (e.g., what is the EROEI and life-cycle CO2 emissions of, say, a 2kW solar PV system with no storage vs the same system with 10 hours battery storage to cover nights [ignoring winter and long cloudy periods])?
5. Lifetime: how many cycles can the storage technology handle (100, 10,000, near-indefinite [e.g. conversion to hydrogen])?
6. Does the storage technology need its own power-generation system, or can it be paired to the original generating technology (e.g., a molten salt heat storage can create steam for use in the same turbine set as the solar thermal plant itself, whereas compressed air energy storage for wind requires a different generation system to the wind itself)?
(If people can propose some other general questions, I’ll add them to this list)
Anyway, to kick the discussion off, here is something sent to me by George Stanford, in response to the following missive:
Seems to me the answer to intermittency is more wind and solar power and larger grids so they have over capacity and can share across larger areas. Conversely consider energy storage devices, flywheel systems, pressure accumulators, batteries, etc. If you consider all the economic benefits of the positive environmental savings of wind and solar (when getting away from coal) then the overcapacity costs would balance out in the end.
The end benefits of ending destructive mining practices, decreased oxides of nitrogen and mercury emissions and disposal issues with fly ash probably make it worth it alone. Has anyone found a study that shows the costs of that.
George replies:
Large-scale energy storage faces hurdles like cost, scale, material availability, and environmental disruption. For example, regarding battery storage, I have found this on the Internet:
Conway, E. (2 September 2008) “World’s biggest battery switched on in Alaska” Telegraph.co.uk, 12:01AM BST 28 Aug 2003
Excerpt: The world’s biggest battery was plugged in yesterday to provide emergency power to one of the United States’ most isolated cities.
The rechargeable battery, which at 2,000 square metres is bigger than a football pitch and weighs 1,300 tonnes, was manufactured by power components specialist ABB to provide electricity to Fairbanks, Alaska’s second-largest city, in the event of a blackout.
Stored in a warehouse near the city, where temperatures plunge to -51 degrees Centigrade in winter, the battery will provide 40 megawatts of power – enough for around 12,000 people – for up to seven minutes.
This is enough time, according to ABB, to start up diesel generators to restore power, an important safeguard since at such low temperatures, water pipes can freeze entirely in two hours. . . .
The earthquake-proof contraption contains 13,760 NiCad cells – bigger versions of those used in many portable electronic appliances including laptop computers and radios. Each cell measures 16in by 21in and weighs more than 12 stone [168 lb].
Notice that this system will store 40 MW x 7 minutes = 280 MW-min of energy. So let’s do a little ball-park arithmetic. The US uses roughly 500 GW-hr of electrical energy per hour. Suppose we want enough storage to supply 1% of that energy for 10 hours (feel free to plug in your own guesses). That’s 50 GW-hr, and 3,000,000 MW-min / 280 MW-min = 10,714.
So for our postulated backup system, multiply the Fairbanks numbers by 10,700. This gives us:
Area: 21.4 million sq. meters = 21.4 sq. km.
Weight of whole system: 1,300 tonnes x 10,700 = ~ 13.9 million tonnes
Weight of NiCad cells= 13,760 x 168 lb x 10,700 = ~ 12.4 million tons = ~12 million tonnes.
Weight of Cd: ~ 3 million tonnes (this is a guess).
World reserves of cadmium: 0.6 – 1.8 million tonnes (inadequate for just US need).
In other words, current battery technology would be unable to support battery storage for any significant part of the electric supply. Equivalent considerations apply to the other storage techniques.
Without storage or hydro backup, wind power looks hopelessly impractical as a major supplier of electrical energy. Larger grid? Apart form the elaborate new transmission network needed, remember that it’s not sufficient merely to always have power being produced somewhere — you constantly have to have enough power to supply the whole grid. Think of the over-capacity needed to accomplish that! Much of the time, most of the windmills would have to be feathered to prevent over-production.
Here-s a year’s worth of daily wind energy from all the wind farms in Germany:
(The Web site I got the above chart from seems now to be inactive.)
You can see almost-real-time info about the wind contribution to the Bonneville Power Authority here:
As for ending the destructive mining practices, etc., that can’t be done more than partially (if that) by wind and solar power — but it certainly can be done, in spades, by fast reactors such as the IFR.
———————-
Then there is this comment by Tom Blees, putting recent solar costs into context (see also my recent comparison in TCASE 15):
This is being cheered on the internet by the windies and sunnies:
The Energy Department on Wednesday approved two loan guarantees worth more than $1 billion for solar energy projects in Nevada and Arizona, two days before the expiration date of a program that has become a rallying cry for Republican critics of the Obama administration’s green energy program.
Energy Secretary Steven Chu said the department has completed a $737 million loan guarantee to Tonopah Solar Energy for a 110 megawatt solar tower on federal land near Tonopah, Nev., and a $337 million guarantee for Mesquite Solar 1 to develop a 150 megawatt solar plant near Phoenix.
The loans were approved under the same program that paid for a $528 million loan to Solyndra Inc., a California solar panel maker that went bankrupt after receiving the money and laid off 1,100 workers.
These people can’t do math. A solar power plant rated at 110MW will have a capacity factor of about 18% tops (because of when the sun shines). Less in winter, of course. So that’s effectively about 20MW for $737 million. The price per gigawatt, then, is about $36 billion! And people complain about the high cost of nuclear power?! Even at the ridiculous prices for new nuclear in the states of about $6-8 billion/GW (and mind you, that’s for 24/7 availability, unlike solar), this solar investment is patently ridiculous.
Edit: It was pointed out in the comments that with Tonopah’s projected capacity factor of 55 % (with energy storage), the capital cost comes to a bit over $13 billion per GWe average power.
Filed under: Nuclear, Renewables
Just posted about exactly this topic in the “CEDA report” thread. I may want to repeat some of that here.
But first, let’s just all agree that having storage is useful for nuclear energy as well as for renewable energy. I recall that the world’s first pressurized air power plant at Huntorf was built to work with a nuclear power plant, switching supply to time zones with better prices and providing backup in case of a station blackout emergency.
http://en.wikipedia.org/wiki/Compressed_air_energy_storage
That kind of storage would have been extremely useful in Fukushima.
//3. If the storage technology becomes cheap, what is to stop baseload plants like coal and nuclear from undercutting renewables, given that they can charge large batteries in low-demand times and then sell the power during peak (high-price) periods?//
This is a great point! I was personally quite amazed at the Nullabor seawater dam claims that a 7km diameter 20m deep dam could store enough energy to run the whole of Australia for 10 hours. The author of the paper said it might come in at $2 billion if the base is not concreted… more if it was concreted.
http://energy.unimelb.edu.au/uploads/Australian_Sustainable_Energy-by_the_numbers3.pdf
Let’s call it $3 billion with concrete.
That’s still cheaper than many baseload nuclear power plants, and how many of those would we need to run the *whole* of Australia for 10 hours? So I was left wondering…. (in my rather scientifically illiterate manner) just how many of these hydro dams we would have to build to have true baseload power from a mix of wind and solar thermal? Add in the cost of the HVDC grid to get the power from the wind across to the Nullarbor and back again. Even so: $3 billion dollars to store power for the whole of Australia for 10 hours?
Ultimately it comes back to point 3 above. If these things are cheaper than building more nukes to cover just a few short periods of peaking power supply each day, then surely the answer is not to try and supply baseload power from intermittent renewables like wind, but shave the costs off the peaking power demands to create even cheaper electricity.
So that maybe, just maybe, we have the money to cope with manufacturing all synfuels we need for airlines etc in a post-peak oil world.
Here is a US DOE presentation that lists a number of current storage projects, the technologies and the varying roles that storage may play.
http://www.iea.org/work/2011/storage/Item2_USDOE.pdf
Beacon Power, the company mentioned as doing flywheel storage for grid frequency stabilization applications has just declared bankruptcy:
http://news.cnet.com/8301-11128_3-20127778-54/flywheel-storage-maker-beacon-power-declares-bankruptcy/
EN I looked at my saved copy of the Seligman pumped seawater proposal and the key data I got out of it was 200 Gwh storage for $35 bn. The 7km diameter octagonal tank (a big version of the one in Okinawa Japan) sits atop a cliff. It was $2n for the tank and $33bn for the pumps and turbines per Table 1. I think this is the same report
http://www.ret.gov.au/energy/…/cei/acre…/029-ProfessorPeterSeligman.pdf
The table says 200 Gwh if I read it right but I haven’t checked the calcs. Other numbers in the report are for generating capacity which we can take as a given as it could just as easily store excess fossil and nuclear baseload for use in peaking.
$35 bn for 200 Gwh is $175 per kwh, similar to lead acid batteries, still the cheapest form of portable energy storage after a century. I seem to recall someone saying that some Army land on the Illawarra escarpment near Wollongong would be ideal for a trial project. Any pilot project needs to be at least a few Gwh to be serious.
Some things to like about pumped seawater include the ready made lower reservoir, no platypus to upset with changing water levels, less drama if the whole thing bursts and minimal electrochemical deterioration unlike a battery.
Just to provide some perspective on what kind of scale we’d need energy storage, this excellent article on The Oil Drum is very useful:
http://www.theoildrum.com/node/8237
The financial and natural resources required are simply prohibitive on this scale. It is interesting that the author of the article puts a careful conclusion in, but any reasonable thinking person will conclude that we simply won’t do this. The risk, then, is that we’ll build some wind and solar and then just run most everything of the remainder on fossil fuels with no end in sight.
This is a risk of fossil fuel lock-in, where it doesn’t matter how cheap wind and solar are, it matters only that they can’t deliver most of the time, and fossil fuels must jump in.
“Notice that this system will store 40 MW x 7 minutes = 80 MW-min of energy”
Can someone show me the math behind this?
Regarding seawater pumped storage cost, I’d caution people from using too optimistic analysis. There is a real seawater pumped hydro station in Japan, 30 MWe, costing 30 billion Yen, which is over 300 million USD, $10/Watt. They had to spend 10% of that just for environmental remediation – this is not a low environmental impact technology. This project stores only a small amount of energy, much more would be needed to truely run on wind and solar.
http://www.ieahydro.org/reports/Annex_VIII_CaseStudy0101_Okinawa_SeawaterPS_Japan.pdf
http://www.iwes.fraunhofer.de/de/highlights/_power-to-gas_-_erneuerbaresgasverbindetenergienetze/_jcr_content/stage/linklistPar/download/file.res/2010-2011_IWES_IB-HL_19%20Power-to-Gas_Erneuerbares%20Gas%20verbindet%20Energienetze.pdf
In Germany, they are proposing to use the gas grid to store variable renewable energy in chemical form.
Excess electricity would be used to produce hydrogen, which would be combined with CO2 derived from biomass to form methane, which would be fed into the gas grid.
The round-trip efficiency of the process is 35%. That’s not good, but it is the only large-scale storage option you have in Germany. According to the authors of the study the technology is supposed to become economically competitive once oil hits 220$ per barrel.
Of course baseload nuclear power plants would produce this “renewable gas” more economically than variable renewable energy sources.
Xoc, thanks for picking up that error, I’ve updated George’s numbers accordingly. Looks better, but still not great…
Molten salts are being used in CSP plants. For example, in Andasol with 2x50MW gross output with about 42% capacity factor (http://en.wikipedia.org/wiki/Andasol_Solar_Power_Station) the storage can provide 7.5 hours of power. Looking at the numbers it seems that one needs around 75 tonnes of salt per MWhe. This implies about 28000 tons for the Andasol power plant. The salts are a 60/40 mixture of sodium and potassium nitrates.
The energy density is in the 50kWh/m^3 ballpark or roughly 20 Wh/kg. This is less the energy density of Li-ion batteries, but not tremendously less than for some other batteries. This is perhaps bit surprising. Sodium nitrate costs about 800 dollars/ton (http://www.indmin.com/Article/2124417/Sodium-nitrates-prices-stagnate.html). For potassium nitrate I cannot find a good source, but in my notes I have a figure of 1000 dollars/ton. This suggests a cost of around 30 dollars/kWh from the salts. Online I can find a total cost estimate of 50 dollars/kWh which seems consistent with my estimate.
(http://www.scientificamerican.com/article.cfm?id=how-to-use-solar-energy-at-night) Another way to state this is that the cost of salts for storage for 7.5 hours adds about 1 billion/GWe delivered to the costs of the power plant. (Naturally 7.5h is not sufficient if our intent is to get rid of fossil fuels.) The amount of storage is set by the heat capacity and volume. I do not believe any major improvements can be expected in this technology.
I can also find a mention that the sodium nitrate peak production has been 3 000 000 tons/year. (http://www.scribd.com/doc/30122669/Sodium-Nitrate-and-Nitrite) If all this production were to be used for CSP storage this rate implies a maximum construction rate for Andasol type plants of 8GWe peak/year. This is almost zero so the global nitrate production would have to be ramped up drastically. I do not know about the reserves for these minerals.
Jani, thanks for those calculations.
The lower energy density isn’t surprising, as sensible heat storage (molten salts) has a lower energy density than chemical change (batteries). Heat is only vibration, whereas chemical change can access the bigger energy levels of the electrons.
There are some major improvements possible.
I should point out firstly that the energy density per liter of salt can be improved by a factor of around 3, by going for a higher temperature. This is very difficult with concentrating solar for cost and thermal loss reasons. But it is easier with a nuclear plant such as an IFR or a LFTR, plus the heat storage system can be used more productively and reliably.
Another improvement is the use of an inexpensive filler, such as sand, in a thermocline system. One of the best easy to read references is this one by James Pacheco:
http://www.p2pays.org/ref/22/21032.pdf
As we can see, the thermocline has theoretically a much lower cost, about 1/3 lower. This assumes a delta T of 84 degrees Celcius, but with a high temperature nuclear reactor up to 300 degrees Celcius temperature drop is possible. This reduces cost of the salt by a factor of 300/84= 3.6. The cost of the tank goes up slightly (need more expensive stainless steels), but the net effect is a big factor reduction in cost, down to around $10/kWh. Even with a bigger salt cost per kg that might happen from increased demand, the cost would be low enough for half a day of storage to deal with daily peaking needs.
I was hoping to use such systems in conjunction with high temperature nuclear reactors so that there would be an integrated baseload-peaker facility with a dedicated peaker turbine sucking on heat from the thermal store. This allows the reactor to operate at full throttle continuously.
“3. If the storage technology becomes cheap, what is to stop baseload plants like coal and nuclear from undercutting renewables, given that they can charge large batteries in low-demand times and then sell the power during peak (high-price) periods?”
There are basically two ways to stop coal (I am not interested in stopping nuclear).
One is what is happening right now in Germany. Renewable energy has priority regardless of price. The feed-in tariff law does not only guarantee that renewable electricity is bought at fixed rates, it also guarantees that every kWh generated displaces exactly one kWh of fossil fuel electricity (Article 8, Paragraph 1 EEG).
Of course one might want to take that a step further and make any use of fossil fuel for any purpose completely illegal, once more people understand the extraordinary dangers associated with said use.
The other is that renewable energy would be just cheaper anyway than coal. Wind is almost there, and solar photovoltaic will be there in less than a decade.
Now, if I’ve calculated it correctly from the Pacheco reference, the thermocline needs 11 tonnes of salt per MWh electrical.
With the higher delta T of 300 degrees Celcius possible with a high temp nuclear reactor, the amount of salt would naturally be reduced by that factor of 3.6, to about 3 tonnes of salt per MWh.
Are you excluding discussion about ‘virtual’ (?) energy storage? I believe there are already companies that are selling commitments to stop using electricity at times of peak demand. I wonder how big this capacity is.
At a smaller scale smart-grid technology can also smooth demand. What are the untapped potentials of these technologies?
There is an interesting suggestion from the University of Minnesota to use the electricity from wind power to generate Ammonia instead of putting the electricity on the grid. This eliminates the problem of connecting the wind farms to the grid and problems with the intermittency of wind power. I’m mostly interested in using Ammonia for vehicle power, while they want to use Ammonia for fertilzer, but it works either way.
http://renewables.morris.umn.edu/wind/ammonia/
OPatrick, on 14 November 2011 at 12:30 AM said:
Are you excluding discussion about ‘virtual’ (?) energy storage? I believe there are already companies that are selling commitments to stop using electricity at times of peak demand
In the US and Canada the Aluminum smelters are for the most part on ‘interruptible power’ agreements. It’s not intended as a ‘daily peak’ but a short term ‘seasonal’ peak. I.E. Summer heat waves.
Alcoa has similar agreements in place in Australia.
http://www.alcoa.com/australia/en/info_page/Energy_Victoria.asp
@Cyril R. Thank you. You are right. Higher temperatures would naturally imply more storage as well as higher efficiences. I have to learn more about thermocline storage systems.
So Harry raises an important point…one that works against, IMO, storage for unreliables.
One is load storage for peaking and before/after peak, a place to put excess daily capacity and use it when its needed.
The second are seasonal or long term issues confronting only unreliables like wind and solar where there could be whole periods of days and even weeks where the sun doesn’t shine or the wind blows.
The BPA situation has been parsed here before so it doesn’t need review.
To get the “24 hour” CSP output, name plate capacity itself drops down tremendously. It’s only good for that ‘day’, so to speak. The same would be true for any massive molten salt storage system, which, I might add, is not being planned anywhere in the world that I know of…because it’s so damn expensive. Still, really good for only about 16hours in even really good insulated vaults.
The German hydrogen thing. The inefficiency is just staggering with the costs involved and the inability to hold a lot of H2 over a long period of time.
Long term storage means pump storage. I can tell you that there IS a lot potential for this in the United States as none of the big hydro projects, about 10 to 16% of US generation, has any form of pump storage. This could be engineered into existing hydro units.
The bottom line, however, is as someone up in the comments section noted *every* form of storage works *better* with nuclear than unreliable wind and solar. It just does. Additionally, when we get to Gen IV deployment even in the upper single digit percentages of grid generation, with their rapid loading and unloading, building to over capacity slightly will eliminate the need for storage altogether.
just to add to Cyrill’s point above…indeed, molten salt storage at high temperatures can be designed integrated into LFTR and IFR simply by adding structure for high temp salt inventory in the secondary loop either at build up or via process heat bleed off and “added modularly” when ever so long as the secondary loop has the ability to off-load hot salt with piping and pumps. I think the potential for this is huge.
Additionally, if we have a “Gen IV Economy” we won’t need any storage as we wont be required to have because there will be no over generation at all. For nuclear energy generally it’s a convenience, for unreliables, it’s a necessity as they can’t control generation, or at least not well enough to make it financially viable to do so.
“The bottom line, however, is as someone up in the comments section noted *every* form of storage works *better* with nuclear than unreliable wind and solar.”
David Walters, isn’t the point more that one of the big perceived disadvantages of renewables – solar and wind – is that they need back-up? If there are other concerns about nuclear then we might overcome these if there is no viable alternative, but with effective energy storage those renewables would be more viable.
Also further to my question above, what price would need to be put on *not* using a kWh of electricity for it to become attractive as an option on a large scale? Could this be managed?
Yes, this is a dumb article (to put it in scientifically technical jargon). Nobody needs (or is working towards) a 7 day battery to store the entire grid. The technology is not there for doing this, it would be hugely impractical and expensive, and there is no need for doing so (is our grid really that much of a failure)? Citing that wind may not blow for a 4 day consecutive period as a justification for such a battery (or thought experiment) shows a serious misunderstanding of the nature of wind power, distributed generation, and the design and operation of energy systems in general.
A couple of sources may help us understand a few features of the problem.
Economic cost of outages? In the US, Berkeley Labs placed this cost at around $80 billion/year (to businesses and consumers, mostly industrial and commercial sectors, $1.5 billion in residential losses). 2/3 of the impact came from momentary (or less than 5 minute) losses. “Momentary interruptions were responsible for two-thirds of the cost, at $52 billion, while sustained interruptions of five minutes or more cause $26 billion. ‘This finding underscores the fact that, for many commercial and industrial customers, it is the length of the ‘down time’ resulting from a loss of power that determines the cost of interruption, not necessarily the length of interruption itself’” (here).
DOE targets for bulk storage in the US (to improve grid reliability, support anticipated expansion of renewables, and prevent economic losses from outages)? In a Feb 2011 planning document, they suggest 10 to 100 GW of bulk storage over the next 5-10 years is a good estimate. For current EES grid targeted batteries (redox flow, Na-based, lead-carbon, Li-ion) they see the following price and performance targets: $1,750/kW capital cost, $250/kWh or less system capital cost, 0.20/kWh levelized cost, 75% system efficiency, 4,000 cycle life. They envision higher targets for new technologies based on previously discovered materials and chemistries: $1,250/kW capital cost, 5,000 cycle life, 80% system efficiency, $150/kWh system capital cost, and 0.10/kWh levelized cost (which would represent some 30% reduction on current costs for many technologies).
A 2009 Arpa-E energy storage workshop has a pretty good summary of current grid energy storage technologies, costs, and benefits. Among those topping the list with detailed descriptions: pumped hydro, CAES, vanadium redox and zinc-bromine flow batteries, lead acid, sodium sulfur batteries, metal air batteries, flywheels, ultracapacitors, and superconducting magnets (SMES). There are also several high-risk concepts mentioned: fuel as storage media, electrochemical conversion to methanol, ericsson cycles, algae farms growing carbon electrodes, multiple platforms V2G, molten salt systems, and more.
Let’s take DOE benchmarks and apply them to economic costs of outages (Berkely Labs study):
– 100 GW bulk storage in 10 years (high end)
– capital cost (best case): $175 billion (over 10 years)
– capital cost (pumped hydro alternative, at full 100GW): $225 billion ($2250/KW, 100-1000MW range, discharge 4-10 hours).
– Benefits of storage (low cost compared to outage costs in Berkeley study): 22% (best case) or 28% (if 100% from pumped hydro at current cost).
– Approx physical dimensions 100GW NaS battery (based on current production NGK 8MW units for daily load shifting): 9,000 square miles (14,500 sq km) or 75,000 cubic km. This is about an area equivalent to Real Energy’s northern Cooper–Eromanga Basin oil and gas and tight gas leasing tenements in Queensland, if units built to 5m height.
– Approx physical dimensions 100GW pumped hydro reservoir (based on multiples of Ludington Storage Plant at 1872 MW): 70 square miles (113 sq km).
There are numerous ways to cover this additional cost of storage for utilities and consumers. DOE energy storage planning document projects EES energy costs in best case will be $0.10 – $0.20/kWh USD (levelized retail rate). At this price, energy arbitrage is an effective way to cover this cost (reducing the overall retail price of electricity at times of peak demand).
Where do you get: “A solar power plant rated at 110MW will have a capacity factor of about 18% tops (because of when the sun shines).”
According to http://upload.wikimedia.org/wikipedia/commons/2/2c/Us_pv_annual_may2004.jpg, a flat plate panel at latitude tilt should get over 25% of peak insolation on average. If this is a solar tower with two axis tilt mirrors it should be even higher.
Have we started comparing apples to mexican jumping beans (and don’t even have an orange on hand to make a faulty comparison)? Solar has no fuel cost, which significant lowers it’s levelized cost of energy when compared to coal, natural gas, or nuclear. Capital costs are reported as a cost for a unit of capacity (not energy delivered). The Crescent Dunes or Tonopah solar tower project is projected to have a 52% capacity factor. It has already secured a power purchase agreement from NV Power for a reported $0.135/kWh (same source). This blog is going to become totally irrelevant if you don’t start giving “fair” representations of competing technologies to nuclear?
I think it is important to understand that there are several different scales of energy storage, and they serve different purposes.
1) Very short time scale storage (circa 1 hour). This serves to smooth out rapid fluctuations in renewable generation (such as wind gusts or clouds). This poses engineering difficulties because the fluctuations are quite fast and large: physical systems like pumped storage may well find themselves putting energy into the reservoir at the same time as they are taking it out. I would expect this smoothing to be done with batteries/capacitors which might, just maybe, be doable for a large renewable contribution (see above battery materials estimates). If you have this, then renewables can be used above the perturbative level. Currently provided by the fuel tanks of peaker plants.
2) Day-length storage (18 hours). For solar, this serves to eliminate the day/night cycle, and an absolute requirement for large solar contributions. For wind, this averages over individual storms and the like. Right now, this can only be provided by pumped storage, or, being honest, fuel tanks. But the duration is short enough that pumped storage can be contemplated. Probably with a shudder. If you don’t have this, the maximum renewable contribution will be really low.
3) Week(s) length storage. This serves to smooth out weather systems. Basically only possible through fuel tanks. Here there is an IMPORTANT thing to realize: my weather right now is correlated with your weather in the past, and that other guy’s weather in the future. In other words, we can claim week(s) length storage, OR geographic smoothing. We can’t claim both at full value. Geographic smoothing is cheaper (unless you use fuel tanks, which is what we do now). If you don’t have this, the maximum renewable contribution will be merely low.
4) Month length storage. This serves to smooth out long term signals, like the factor of near 10 in Germany’s summer/winter solar output. Required for solar to be useful at any significant latitude. Only possible with fuel tanks. This, finally, allows for a high renewables contribution. Pity we can only do it through fuel tanks…
Funny, I said “fuel tanks” a lot. Non-fuel tank solutions are really only available for (1) and (2).
DOE…In a Feb 2011 planning document
In a Feb 2011 Research and Development Goals Document
http://energy.gov/sites/prod/files/oeprod/DocumentsandMedia/OE_Energy_Storage_Program_Plan_Feburary_2011v3.pdf
Under ‘Challenges’ on Page 17
Challenges—Most of current EES technologies are not competitive in capital cost and/or life cycle cost for broad market penetration.
Challenges—As a utility asset, storage technologies are required for a life at least 10 years, often longer, and a deep cycle life (e.g., more than 4,000 cycles). Minimum or no maintenance is preferable. Long term reliability and durability are not typically proven for technologies entering the market.
Challenges—The non-vehicle energy storage sector is still, generally, commercially immature.There is not enough manufacturing capability, particularly in the U.S., to deliver the projected quantity of EES systems to meet future demands.
Then on page 28 as a ‘goal’
A national assessment of the role of energy storage: This will quantify the role of energy storage technologies as the grid transitions to a greener, reliable, and highly efficient energy infrastructure. The assessment will estimate the potential market size of energy storage for various grid services as storage competes against other generation technologies (such as gas turbines), demand response, and transmission assets.
Page 33 – an improved cost modeling system
Detailed cost and “state of health modeling” of energy storage: To provide transparency of the cost composition of an entire battery system, component-based cost models will be developed for
stationary energy storage systems.
Here is a US DOE discussion of ‘fusion’
http://science.energy.gov/fes/
With the support of FES, a devoted, expert, and innovative scientific and engineering workforce has been responsible for the impressive progress in harnessing fusion energy since the earliest fusion experiments over sixty years ago. As a result, we are on the verge of a new age in fusion science during which researchers will undertake fundamental tests of fusion energy’s viability.
EL, you’re not listening to what I’m saying.
First and foremost, running with less than at least a week of full power storage on a national level whilst relying on unreliables, means burning lots of fossil fuels. In a growing world economy and energy demand, even 25% fossil isn’t acceptable.
Second, the article is good in that it tries to do this. It is not a dumb article, it is extremely useful limit analysis. It does not matter if you insert pumped hydro, the conclusion is the same since pumped hydro has not much lower cost than lead acid.
Third, if your notion of a wind and solar powered world is one with less reliable electricity and longer outages, then just be honest about that.
Here in the Netherlands, a single 5 minute outage per year is considered a national scandal. Good luck eating away some nines in reliability – my reliability last year was 100%, same as the year before. Not 99.9999 percent, 100 percent. Zero outage. Not one second. I’m willing to pay for this. I’m not willing to pay a lot of money for greenwashing fossil fuels with unreliables. I have little patience for energy plans that don’t add up.
Barry,
I haven’t caught up with all the comments on this thread, so apologies if someone else has already pointed this out.
Tonopah has molten salt storage and expects to generate 485,000 MWh per year http://www.nrel.gov/csp/solarpaces/project_detail.cfm/projectID=60 . So the expected capacity factor is 50%. Therefore, average power is 55 MW. On these figures, the capital cost per average power delivered is:
$737,000,000 / 55,000,000 W = $13.3/W.
Also, EL, uranium costs 0.3 cents per kWh. This is not a substantial advantage for PV. PV being a marginal energy source that is not there 80% of the time in the desert, and not there 90% of the time in Germany, gets little capacity credit. So PV has to compete with 0.3 cents per kWh nuclear, or 2-3 cents per kWh coal fuel.
This is why significant buildout of PV only occurs with massive subsidies, several times market rates for an energy source that isn’t worth 1% of that.
By the way, $13.3/average Watt is twice the cost of the most expensive nuclear power plant being constructed right now (Olkiluoto).
Has anyone else noticed these massive internal inconsistencies of the pro unreliables anti nuclear folks? Ask them about nuclear they’ll say, oh the waste, its such a problem. They never look at the waste of the unreliables. They’ll make vague statements about innovation. When asked about nuclear innovation in pyroprocessing and next gen nuclear power they’ll say it can’t be done. But they’ll happily make breakthrough technologies in energy storage part of their energy plans.
The fact that the whole energy system of the world (not only electricity) can be powered for decades from stored energy follows clearly from the fact that it is already done right now.
Fossil fuel is nothing else than stored energy, though admittedly stored by an extremely inefficient process taking hundreds of millions of years and provided by nature.
Since there is technology available to make many different kinds of synthetic fuel (hydrogen, ammonia as mentioned above, quicklime, methane gas, methanol, gasoline) it is just a question of how much of your carbon free capacity (nuclear or renewable, doesn’t matter for storage) you want to dedicate to making those fuels. That is a question the market will figure out.
I recall that the “Energy from Thorium” blog just discussed some such schemes in connection with nuclear energy:
http://energyfromthorium.com/2011/11/07/nuclear-cement/
If you take the CO2 necessary for making synthetic fuels right out of the air as the “Green Freedom” project described at the link proposes, there is nothing to stop you from taking twice as much CO2 as needed and dispose of half of it (by weathering peridotite or olivine), making CO2 negative fuel.
The fact is that most hydrocarbon fuels had several hundred million years preparation time. As that carbon was buried the atmosphere’s oxygen content increased. Very late on the scene humans worked out how to exploit this. Now we want fuels produced in real time, not in batches over millions of years. It’s as if we were fleas infesting a dog and now we’re saying ‘this dog is sick let’s find another one’.
Karl-Friedrich Lenz,
it is just a question of how much of your carbon free capacity (nuclear or renewable, doesn’t matter for storage) you want to dedicate to making those fuels.
If one isn’t careful the Energy Returned on Energy Invested ends up being less then one.
More pumped hydro and other storage technologies
http://www.dotyenergy.com/PDFs/Doty-90377-Storage-ASME-ES10.pdf
http://www.electricitystorage.org/technology/storage_technologies/technology_comparison
In particular, the Doty Energy study suggests that even ordinary pump hydro for diurnal cycling is fairly expensive. Of course, for that the geography (and other factors) has to be appropriate. Underground pumped hydro can be built almost anywhere, but the costs are then much higher.
Unless the unreliables become nearly free, using those to energize a storage scheme is not economic.
Following
I had a chance to review several storage schemes as part of a DOE evaluation team. Most of the schemes had approximate costs of $1 per watt for the equipment and $0.40 per watt hour. Redcently I went to a luncheon talk on storage and noted the price of their equipment went up to $1.2 per watt. The battery cost was not stated. I think these numbers are good for rough estimates.
Cyril R., on 13 November 2011 at 9:27 PM – where did you get the costings for the Okinawa pumped storage plant from?
@harrywr2
I don’t understand that. Obviously, any form of storage will come at a loss compared to just using electricity as it is produced. But if you want to time-shift your energy, or use it in a car where you have no way of delivering it except carrying it with you in some way, that can’t be helped.
This is not about EVs but about “time-shift” energy (i like the term) for purposes of using it later either:
1. Because you *need* it later or
2. You have too much now and can’t use but could use it later.
Yes there is a loss for storage. So the question is, then, why are we storing generation? Is there any financial, resource utilization advantage to doing this? If we add storage to CSP do we not have to knock down the actual name-plate capacity by a factor of 4 or 5?
DW
David Walters — The nameplate (MWe) is provided by the actual generator; the thermal source provides MWt which with some efficiency and possible delay via thermal stoarage then runs the generator.
To compare the cost of unreliables + storage with nuclear, do the comparison on an LCOE basis. For unreliables add the capital cost of the unreliable generator plus the capital cost of storage.
You may need to replace the storage several times during the life of the unreliable generator, so you need to include multiples of the capital cost of the storage.
You will need to replace the unreliable generator and generator several times during the life of the nuclear plant. So you need to include multiples of the capital cost of the unreliable system.
The capacity factor for nuclear is normally quoted is the expected life time capacity factor for the system, allowing for planned and unplanned outages. However, the capacity factor for the unreliables is normally quoted as the best that could possibly occur over a short term, rather than the average over the plant life. Therefore, the unreliables’ energy output and capacity factor should be the expected life time values (to be valid, these figures should be based on actual experience from actual plant lifetimes and performance).
In calculating the LCOE of the unreliable system, you also need to add the cost per MWh of energy storage capacity.
If the proponents of unreliables would do these calculations themselves, they might start to realise why most people why have already done such calculations realise that unreliables are a massive waste of time and money. They are a distraction.
I just read the link given upthread by harrywr2 on the views of heavy industry like aluminium smelting. They are a bit disingenuous saying they got brown coal fired electricity cheaply so as to use up capacity. That’s a bit like eating bad food so as not to waste it. They seem as bamboozled by carbon tax as everybody else. Note hydro + nukes = no problems for aluminium apart from some perfluorocarbon emissions.
Their views on intermittent power are revealing. Again the link is
http://www.alcoa.com/australia/en/info_page/Energy_Victoria.asp
@David Walters
Storage is very much about electrical vehicles. That is where a lot of the deployment will happen and will be necessary. Regardless of your carbon free energy source, you will always need some way of storage to carry the energy with you in a car. Same with airplanes.
This might also be a good time to quote David McKay, who has shown that the amount of storage needed per person “beautifully matches” what will be available for free anyway once everybody switches to electrical cars:
http://www.inference.phy.cam.ac.uk/withouthotair/c26/page_195.shtml
The economic case for adding storage for time-shifting right now is that this is the only way to use electricity if you generate more than supply. If you don’t have hydrogen storage, as the Enertrag hybrid wind park recently opened in Germany has, you would need to just turn off your generation whenever the grid can’t handle the extra load.
Reference for Enertrag hybrid wind park:
http://k-lenz.de/1004 (Lenz Blog)
The same is true for running a nuclear based system like in France. If demand drops, you need to throttle or completely turn off your nuclear power plants if you don’t have storage. Again, storage is useful and for cars essential regardless of what flavor of carbon free generation you prefer.
People want storage right now because there is too much electricity in certain time slots and they don’t want to throw that away. Alternatively, they want time-shifting since it enables them to sell their power when prices are highest.
KFL
The role of EV’s will most likely be restricted to limited use of batteries for occasional high demand days. Given the high cost of EV batteries and their cycle-limited life, consumers will need an exceptional premium from network operators to justify limiting the life span of their batteries, providing they are available at peak times. The use of EV’s will be primarily for converting baseload to peak load, rather than converting intermittent to baseload. This seems to be a widely misunderstood by renewable advocates. The IEA Smart Grid Roadmap briefly discusses these issues:
http://www.iea.org/papers/2011/smartgrids_roadmap.pdf
Barry:
Peter Lang:
The capacity factor is not such a simple metric when storage is involved. For a CSP project like this you can meaningfully talk about the capacity factor of the solar field, or the capacity factor of the turbine. If you try to define the capacity factor of the integrated facility, it can only be relative to some nominal “expected” output, which may be arbitrarily chosen, and therefore not very meaningful.
Lets look at this Tonopah facility. From Peter’s link it has a 110 MW turbine which is expected to produce 485,000 MWh/y. So the turbine is operating at 50% capacity factor (55 MW continuous).
But what’s the solar field? From SolarReserve’s FAQ,
The plant will generate between 100-600 megawatts of electricity, depending on the configuration of power load that the utility or customer requires.
So it looks like the solar field has ~600 MW capacity. If Barry’s 18% capacity factor applied that would be an average 108 MW continuous equivalent.
But the expected continuous equivalent is only 55 MW. So the solar field is operating at only 9% capacity factor. Thats not much.
I assume the drop from 18% might include poor winter output, round trip efficiency from collector to store to turbine, and thermal losses from storage, particularly if more heat energy is stored than used in a single night – that excess is probably lost.
So you can argue this facility has a capacity factor of 9%, or 50%, or anywhere in between. Its a configuration tradeoff. You could give it a 100% capacity factor if you liked, just give it a really small turbine. A high capacity factor is meaningless by itself.
@Graham Palmer
McKay doesn’t address questions of how the market will play out, he only points out that 30 million electrical vehicles will provide 1200 GWh of storage.
Even if they won’t feed energy back to the grid, this is clearly a massive amount of storage. If that can be done, then 50 GWh storage as assumed to be impossible in the post above should be not much of a problem as well.
And even if you want to build your system with nuclear only (good luck with the politics of that), you would still need batteries or synthetic fuel for all the cars. There is no way around building these massive storage capacities.
Actually, those capacity factors suggest a useful metric:
Generator CF / Collector CF = Overbuild required for realized output
ie for Tonopah to realize 485000 MWh/y (55 MW equivalent) from a 110 MW turbine it had to build solar capacity of 5x the turbine rating.
That would be a more meaningful way to compare different CSP facilities and possibly other stored energy systems. Smaller overbuild is better, as it reflects storage efficiency and appropriate turbine sizing.
John Morgan, yes, excellent points. Still, the original estimate of the cost over average delivered power was clearly wrong… putting aside issues of the quality/dispatchabiliy of the electricity generated from Tonopah.
[…] Joel Upchurch offered a BNC comment that caught my attention — connect unreliable wind not to the grid, but to anhydrous ammonia plants: […]
Karl, yes electric vehicle charging is a big energy sink. However it is mostly a day to day energy sink, most cars being driven daily. So it is useful for nuclear, that has excess nighttime capacity for people to charge their vehicles when they sleep. It is not useful to deal with longer term wind lulls and not useful to deal with solar (which isn’t there in the evening and nighttime when people come home and want to charge).
Electric vehicles are an important enabler for a close to 100% nuclear grid, as most mismatch is diurnal and this is taken care of with the controllable charging rate of electric vehicles.
Clearly electric vehicles are a much better match to reliable nuclear power output than unreliable wind and solar.
Hi John Morgan,
The ‘solar multiple’ is set so the solar field provides sufficent energy over the day time to charge the storage for the number of hours of generation at full power stated. I don’t recall if this is based on annual average charging hours or winter charging hours.
Let’s assume we have 8 hours when the solar plant can generate electricity at full power. If we want 8 hours of storage as well as generating in the day time we need a solar multiple of 2. If we want 16 hours storage we need a solar multiple of 3. Because the distance from colletor to power tower of power block increases as the solar multiple increases, the cost increases faster than the storage capacity. You may recall the NEEDS analysis explained this well.
http://www.needs-project.org/RS1a/RS1a%20D12.2%20Final%20report%20concentrating%20solar%20thermal%20power%20plants.pdf
I think the capacity factor refers to the number of hours per year the plant can generate at full power.
However, I’d add for the benefit of other readers, the capacity factor of an ‘unrelable’ is not equivalent to the capacity factor of a fossil fuel, hydro or nuclear plant. The capacity factor of the reliables is determined by their availability and the amount they are dispatched by the operator. The capacity factor of unreliables is generally the best you could hope for and is not a realistic indication of their average life time capacity factor.
Jani Martikainen
Sodium nitrate is rarely mined anymore; it is made.
There are several industrially important processes for making sodium nitrate.
The nitrate ion invariably comes from haber-bosch in all of these processes(either as nitric acid or ammonium nitrate, depending on which process is used). Haber-bosch uses any source of hydrogen, heat and pressure to make ammonia(usually ‘stranded’ natural gas is used), which can be oxidized further to nitric oxide, which react with water to produce nitric acid.
The sodium is used as either sodium hydroxide, sodium bicarbonate or sodium carbonate depending on process. Sodium carbonate is mined or made from brine and limestone in the solvay process.
The raw materials are abundant but it takes A LOT of energy to make sodium nitrate, mainly because of the nitrate.
@Soylent: Thanks for the info. Do you have any idea how much energy is required?
@ EL, on 14 November 2011 at 3:22 AM.
In a couple of dozen paragraphs about energy storage, EL mentioned only power (kW, MW), not energy (kWh), until the final paragraph. No link between these two measures was made.
Discussions about time-shifting energy must account for both the energy recoverable from storage and the rate at which it is recovered, ie the power. Ditto input power and energy, but the energy figure represents the size of the battery or flywheel or dam or whatever the storage system is, and is thus the first consideration.
In terms of Barry’s questions 1 – 6, Energy is Q1. Power, the rate in kW or MW that energy can be fed back to the grid in times of need, is just as relevant (Q7?). Connections between generation and storage systems, ie transmission capital and operating costs, are worthy of becoming Q9. The results from Q8 and Q9 are needed as inputs for calculation of system (not simply generating unit) LCOE’s. As Peter Lang mentioned above, the system is what matters, not components. He cited the possible need for several sets of batteries to span the life of longer-lived components.
However, this thread is about storage, so I will return to it with a simple rule-of-thumb example.
The power of a hydro system which is adequate as a storage and reserve generation component of a coal or nuclear powered notional 20GW base loaded system might well be about the size of a couple of the largest generating units, say 2GW total (10%). If the same system was adapted to back up a series of wind farms, the maths changes hugely.
Capacity factor considerations suggest that, with wind at say 25%, there must be 80GW of wind turbines to harvest that notional 20GWx8660 hours load, averaged over a notional year to allow for seasonal effects.
When generating, up to 60GW will be sent to storage. The pumped hydro storage system needs 60GW of pumps and use all of that wind… or even more wind turbines, or some dirty GT’s or… this is the point at which the wind sales person may deflect the discussion to considerations of HVDC links circling the earth, or charging batteries on 30 million EV’s at 2kW, or some other equally unachievable non-solution.
Summarising, for a notional 20GW steady load system.
Reliable baseload requires:
Backup plant – say 20% => 24GW installed.
Hydro backup generation: say 2GW.
Hydro pumping back uphill: allow 30% cycle losses = 2 x 1.30 = 2.60GW.
Water storage uphill of say X Gigalitres.
The equivalent wind setup will require:
80GW nameplate plus pump plus 30% cycle losses = 100GW installed.
Hydro backup of 19GW generating.
Pump capacity of 80GW.
Water storage capacity >> 20X gigalitres, depending on how long the summer doldrums need to be fed using water stored during winter’s windy season. I suspect that 100X will not suffice in the real world.
The wind system will also need 80GW connection to the pumped storage facility, compared to 2.6GW for reliable baseload. Both systems require 20GW connection to their loads.
So, it becomes evident that reliability of input energy is the single most valuable factor in any dependable electricity network. I suspect that the same, more or less, applies to other energy types, eg natural gas or batteries, sun, wind, liquids and all the rest. If input energy is less likely to be there when needed, the cost of unreliability rapidly becomes huge due to the need for storage.
@ Graham Palmer,
thank you for providing that link on EV’s. It makes sense. Using EV’s to backup an intermittent grid would probably kill the battery years earlier. We’re going to have enough problems just asking EV’s to reduce even *some* of our demand for oil, let alone asking them to solve a messed up intermittent grid. I’ll stop spreading such rubbish until I actually see some super-battery perform this miracle over decades.
@ harrywr2, on 14 November 2011 at 4:45 AM:
Great citation from the US Dept of Energy. The table of 5-year goals on Page 2 includes target costs which could be taken as a current upper bound answer to Barry’s Questions 2 and 5 and as inputs to indicative LCOE calculations.
The text provides an easily digested overview of much of this thread’s topic.
Bravo!
I’ve got some questions that I’ve not the time to answer. Sorry for that, I’m a commercial scientist with tight deadlines and demanding customers.
I’ve heard talk of compressed air storage using geological structures or tunnels to hold energy. Does this storage hold a future?
Yes, I’ve also run the numbers on the cost of cycling batteries of electric vehicles for grid storage, and concluded it is too expensive for today’s li-ion and LiFePo batteries; it would be cheaper to use lower cost dedicated deep cycle lead acid (which is still prohibitively expensive for bulk electric storage, by the way). If the lithium titanate batteries deliver on cycle life, this vehicle to grid idea could work.
Personally I don’t see the need for storage; simply having the electric vehicles on scheduled demand – controlling only the charging rate without actually discharging for storage – is good enough to integrate close to 100% nuclear.
What Soylent says is very interesting. I thought most nitrate was still mined. If it isn’t then this would indeed be a very big energy sink for an energy storage application. Electrolysis of NaCl and production of nitrate component is very energy intensive. I wonder how it affects energy return on investment for a very large CSP thermal storage that would be needed to cover several cloudy days…
@ Karl-Friedrich Lenz, on 13 November 2011 at 10:52 PM:
When a kWh of renewable energy is produced, it may displace a kWh of FF electricity, but that is not the end of the matter.
The carbon dioxide emissions which are thus avoided means that, under a capped ETS, another similar amount of carbon dioxide may be released elsewhere. Somebody else’s marginal additional carbon emissions will fit under the cap to fill the space vacated by the zero-carbon production. The nett effect of adding extra low- or no-carbon generation in a capped scheme is thus precisely zero. What happens is that there is one more kWh of power available.
Jevon’s Paradox ensures that this additional energy will find a user.
The same is true when Australians opt to purchase Green Power. The green customers pay more for the privilege of helping their retailer to offer a cheaper mix of more carbon intensive electricity to its other customers, whilst still meeting the legislated green energy targets.
The green power users are paying money to support the carbon-emitters, at least until the cap is further reduced, thus forcing the retailer to purchase more green power for sale to the non-green customers. The green power customers have wasted their money.
The only batteries that have truly long life times are redox. I think the Vanadium redox was invented in Australia. They are very suitable for substation use. http://www.google.com/search?q=vanadium+redox+battery&rls=com.microsoft:en-us:IE-SearchBox&ie=UTF-8&oe=UTF-8&sourceid=ie7&rlz=1I7ADBR
@John Bennetts: It is kind of funny that your argument has been used both to oppose wind power as well as by the anti-nuclear crowd to oppose nuclear. The problem is there and is related to the stupid design of the ETS. I guess that the cap should automatically be lowered when ever new carbon free power source comes online, but that is not the case today. The caps are set by politicians who naturally feel also other pressures than those caused by the climate change. Also, only some GHG sources are under the ETS in the European Union. I think that for example district heating is not in the ETS system. So if you have a CHP plant and you need heat, CO2 is still emitted from the plant no matter what renewable sources have a priority access to the grid.
@John Bennets
I have argued against this understanding of ETS in another thread. With exactly the same reasoning you can say that shutting down nuclear in Germany doesn’t matter for the climate since carbon emission levels are fixed anyway. And the government did exactly that.
This does not factor in that the amount of permits in the system are not fixed forever, but may and should be adjusted by legislation, looking at how much carbon emissions are actually needed. I just called for doing exactly that a couple of days ago on my blog, since prices for carbon permits have fallen under 10 Euro per ton, indicating that there are way too many of them around.
I don’t buy the idea that a fixed emissions level makes any efforts at carbon emission reductions unnecessary. If it did, that would be rather counterproductive.
John Bennetts, on 14 November 2011 at 10:21 PM said:
The table of 5-year goals on Page 2 includes target costs which could be taken as a current upper bound
http://energy.gov/sites/prod/files/oeprod/DocumentsandMedia/OE_Energy_Storage_Program_Plan_Feburary_2011v3.pdf
I think we have to take them as ‘goals’ that are not yet realized.
For example one of the goals for 2012 was to complete evaluation of the Beacon Flywheel Storage System.
Beacon went bankrupt 2 weeks ago. In the US Bankruptcy doesn’t necessarily mean ‘the end’.
V2G is not likely to be built around today’s high cost, low energy density, and high material demand battery technologies. The wishful thinking about a 300-500 mile range EV battery is not going to come from conventional Li-ion chemistries, but from metal air batteries. These have much higher specific energies, are inexpensive to produce, raw materials are more abundant than lithium, have low toxicity (for easy recyclability), and are now feasible with technological fixes for cycle life (which was previously limiting factor for this very old and established technology). Car manufactures now are racing to patent their game changing metal air or lithium air battery designs, and technology companies like Google, IBM, and GE want in on the game too. “Eos’ proprietary rechargeable zinc-air battery will initially be sold for $1000/kW and $160/kWh, and is electrically rechargeable with a life of over 10,000 cycles (30 years) with a full duty cycle and at full depth of discharge.” Musk at Tesla believes ultracapacitors will likely win out in the end.
The business model for flywheel storage rests heavily on electricity market regulation, and contrasting costs between “fast” frequency regulation and “slow” fossil-fuel generation. New FERC rules in late October were targeted to address these issues, but came too late for Beacon. “The ruling could have doubled Beacon revenues but time ran out” (here).
One could say much the same for Solyndra. They were undercut by low cost solar panels from China (with some accusing China of unfair trade practices and dumping solar panels on US markets for a loss). Not sure either of these cases has anything to do technological goals “not yet realized,” but more to do with the highly competitive nature and rapid fire business markets for these emerging technologies. And flywheel storage is expensive when compared to more cost effective battery storage, a market that is likely to see $122 billion in investment over the next 10 years (according to
one research report).
@ Paul Whyte:
I’ve heard talk of compressed air storage using geological structures or tunnels to hold energy. Does this storage hold a future?
I’ve seen this proposed before with regard to storing wind-generated energy. I understand it would have some issues, one of which is the massive temperature drop inevitable with depressurisation as the energy is extracted. This would cause ice to form in the exhaust stream which poses threat of damage to the turbine. The proposed solution to this problem was reheating of the air stream with natural gas combustion, which seems to defeat the purpose of low CO2 generation.
EL
“Eos’ proprietary rechargeable zinc-air battery will initially be sold for $1000/kW and $160/kWh
There seems to be some legal discussion about the ‘propriatery’ silicon technology company that shares street address and management as EOS Energy Storage, formerly known as Grid Storage.
http://guntherportfolio.com/2011/03/solar-grade-litigation-dow-corning-vs-rsi-silicon/
I find it difficult to believe that in an industry where 100′s of millions of dollars are being spent on R&D that a single individual working for a privately held firm in Easton, PA could come up with breakthroughs in both Silicon and Battery technology in the space of less then 4 years.
Note about ammonia syntheis for energy storage:
Ammonia is already moved by tanks & pipelines so it might end up generated by stranded hydroelectric & geothermal plants & moved elsewhere in the world rather than being used to even out the swings of wind & solar production.
This would especially be the case if this:
http://www.energy.iastate.edu/Renewable/ammonia/ammonia/2007/SSAS_Oct2007_Final.pdf
becomes as cheap as is claimed in the pdf.
@Jani Martikainen
You can get a lower bound by just looking at the haber-bosch process. The molar weight of ammonia is 17 g/mol; and the molar weight of sodium nitrate is 88 g/mol; so 17 grams of ammonia can be used for 88 grams of sodium nitrate.
Making a metric tonne of ammonia with haber-bosch from natural gas requires ~10 MWh of mostly natural gas. Making a metric tonne of ammonia from electricity with electrolysis and haber-bosch requires ~12 MWh of electricity.
At an absolute minimum you need 1.9 MWh of natural gas or 2.3 MWh of electricity per tonne of sodium nitrate. That’s ignoring transportation, purification and the sodium side of things altoghether.
The generation cost of the gemasolar plant(a solar thermal plant with molten salt storage) is estimated at 27 eurocents per kWh by its owners; If solar thermal had to “eat its own cooking” the absolute minimum cost for just the energy(not to mention capital, labour, transporation…) for sodium nitrate would be €600 per tonne.
Adiabatic CAES (or “advanced” CAES) removes the need for a natural gas burner or heated metal mass, and is expected to have a roundtrip efficiency of around 70%. The following peer-reviewed study envisions artificial systems (where natural reservoirs are lacking) in 1-10 MW range, and from components “widely available on an industrial basis” (p. 11). Currently, the costs of such a system are prohibitive when compared to natural storage plants (such as the 200 MW Adiabatic CAES plant in Staßfurt Germany, which will utilize salt caverns).
The aqueous sodium battery from Aquion looks promising. They’re aiming at a lead-acid price-point per Wh, but with 10,000 cycles or more (currently tested to 5000 cycles). So the long-term capital costs would be 20x less than lead-acid. Materials are cheap and plentiful: water, sodium sulfate, manganese oxide, and carbon.
CAES has been around a long time – in Germany. The idea is to use low incremental cost base load generation at night to charge up the compressed air. Then during the peak of the day the compressed air replaces the compressor stage on a CT. The CT is able to put out a lot more electrical power because it does not have the work of the turbine compressor stage. This is the concept. However when implemented in Germany, the operators wanted to hold the generation in reserve because of its quick start and black start capabilities rather than use it ot move low cost energy from off peak to the peak period so the original concept was not realized in practice. I guess if Germany does not have nuclear plants and coal is being retired, the CAES can now be charged with wind energy. It could have a nice leveling effect for wind. All the CAES I know of use salt domes and very high pressure air. This is necessary to keep the salt dome from slowly shrinking. Houston Light and Power (now Reliant) once looked at CAES but decided against it because the salt domes were more valuable as natural gas storage than as compressed air. So there are many variables to consider when deciding to use CAES or not. The good news is that its a mature technology.
Urban and rural biowaste has the largest capacity for contributing to renewable and and even nuclear energy production- if its converted into methanol.
Converting biowaste into methanol would allow practically any community on Earth to use it for base load or peak load energy production in addition to the added versatility of converting methanol into gasoline through the MTG process for automobile transportation.
Adding nuclear hydrogen to the mix could potentially increase biowaste methanol production five fold. If this were done in America, the US could completely eliminate the need for foreign and domestic petroleum.
@EL
The German pressurized air plans are described in detail in this blog post at the “Smart Grids” blog:
http://k-lenz.de/1005
They say that RWE has succeeded in raising the efficiency of the process from 50 to 70% with the simple idea of storing the heat that gets released when pressuring air. There are plans for 20 compressed air storage plants, with the first 360 MW project to start construction in 2013.
The nice thing about this approach is that the sites suited for pressurized air storage are located in the north of Germany, near to the wind parks. No need to transport the electricity to the mountains in the south for water pump storage.
Karl, your reference project is for a 4 full load hour storage system (360MWh/90MW).
Looking at Germany’s wind turbine output, they need more like, at least 4 days.
http://uvdiv.blogspot.com/2010/03/uptime-downtime_07.html
Looking at Germany’s PV output, 4 months wouldn’t be enough (Germany needs more energy in winter when its cold dark and not sunny).
http://energyfromthorium.com/forum/viewtopic.php?f=39&t=2689
What’s the plan for the longer term backup/storage?
Let me guess: burn fossil fuels.
Karl, you go ahead and be politically correct and physically incorrect. I’ll take my chances with turning over public opinion and politics. If we go against physics and nature, we’ll lose for sure, whereas with politics we have a fighting chance.
John Bennetts, on 14 November 2011 at 8:53 PM said:
You seem to be misunderstanding how pumped hydro works:
Hydro pumping back uphill: allow 30% cycle losses = 2 x 1.30 = 2.60GW.
The losses mean more GWh storage but not larger pumping capacity. Remember that only a portion of total consumption needs to be stored.
The equivalent wind setup will require:
80GW nameplate plus pump plus 30% cycle losses = 100GW installed.
Cycle losses only apply to the fraction of yearly GWh consumption that needs to be stored. Wind turbines connected to the AEMCO grid in SE Australia are generating slightly less than the average capacity factor(0.33) to slightly more than this most of the time. Thus most of the time only a small portion of the 20GW demand will be stored or released via hydro. Pumping losses only apply to this portion.
<Hydro backup of 19GW generating.
this seems correct, but since demand is not a steady 20GW would need additional hydro capacity for peak demand(30-35GW)
Pump capacity of 80GW
In Australia’s case would need 60GW wind capacity plus enough to cover pumping losses plus losses due to wind spill during high wind output(say above 70% capacity). An additional 10% generating capacity should cover these two losses, or 66GW for Australia ( or 88GW if using the 0.25% capacity factor).
Returning to what pumping capacity would be required, if demand is 20GW and >70% is spilled would need (66×0.7=46GW minus 20GW=26GW pumping). If peak demand is 35GW would need 33.5GW hydro generation so could probably have 27GW pumping available. With 0.25 CF would need 40GW pumping, so it would be better to install wind turbines with a higher CF.
The actual GWh needed to be stored would be much higher than that needed for meeting daily peaks using coal or nuclear. With large hydro storage dams this is not an additional cost, the cost is for the pumps and tunnels( ie GW capacity not GWh).
Neil Howes,
(Personal comment deleted)
Pumped-hydro cannot be used economically for back up for wind energy at the scale that would be needed. If divert the little pumped storage potential Australia has for unreliables, it would not available for where it could be used for much higher value. If it was diverted to trying to make unreliables a little less unreliable, that woud be a serious misuse of Australian resources.
Better Place battery swap EV’s were on SBS the other night… I haven’t seen it yet.
http://tinyurl.com/7on3vlj
MODERATOR
Please check BNC’s citation policy before posting a link. You should watch the link and give your opinion on it before commenting on BNC.
@Cyril R.
They are talking about 360 MWh at 20 sites, which adds up to 7200 MWh. Of course that is probably not enough, but it is also only one of many options.
Your question about “my plans” for long term storage gives me a good opportunity to mention the quicklime cycle, as proposed in the recent paper by Benjamin Müller and colleagues at the University of Erlangen quoted here:
http://k-lenz.de/1006 Lenz Blog September 2
They only propose that as a method of transporting energy from the Middle East and North Africa (MENA) Desertec project to Germany, but there is nothing to keep people from building a stockpile of the stuff.
Also, since there needs to be much more electricity generation than demand (to make synthetic fuels for cars, trucks, airplanes and industrial processes, as well as to make and transport water in a hotter world), it follows that one would just burn carbon neutral synthetic fuel on the occasions it is needed. On a small scale that is exactly what Enertrag has started doing right now.
I would also like to recall that I am strongly in favor of keeping existing and aggressively adding new nuclear capacity, so in my energy scenario I have nuclear power stations running all the time at full capacity and mostly making fuel or water. They could be used anytime to fill in. While I think it is certainly possible to go renewable only (Iceland shows that it is already done), I don’t see any merit in excluding any non carbon source, especially a heavy hitter like nuclear.
My plan would certainly not include any use of fossil fuel.
kap55, on 15 November 2011 at 7:44 AM said:
The aqueous sodium battery from Aquion looks promising
http://www.technologyreview.com/energy/38689/page2/
The company is making 35-watt-hour units that are modular and stackable at its research and development facility.
MODERATOR
Please read BNC Citation Rule and provide more analysis.
Tom Murphy has again a wonderful posting in his “Do the Math” blog. This time on pumped storage. http://physics.ucsd.edu/do-the-math/2011/11/pump-up-the-storage/
I think he cuts straight trough to the relevant issues.
MODERATOR
Please read BNC Citation Rule and provide more analysis.
There is no doubt that Germany is on the right track.
We have been a powerhouse of engineering ever since.
Here`s another good idea which does have potential.
http://www.authorstream.com/Presentation/heindl-958931-hydraulic-energy-store-system/
MODERATOR
Please read BNC Citation Rule and provide more analysis.
We’re going to have to try and get a better handle on storage needs for modern energy systems now and in the future with greater degrees of renewable energy penetration. The two sources mentioned above are entirely off base, and have no credible basis in any research done to date (in peer reviewed literature, by technical advisory groups, governments, private utilities, independent operators, ancillary service providers, and the like). Let us try and not side track the discussion into power systems operation and the merits (pros and cons) of variable generation, but just stick to what has been documented, and where the needs stand today and in the forseeable future. Kray has a very useful comment above on scales of energy storage (and their application): 1 hour or less (frequency regulation), 18 hour (load following and backup for variable generation), week and month long (seasonal demand basis).
It seems obvious to me that seasonal load variability is best handled with adequate resource planning and not with storage (especially since we have no storage alternatives to handle large fluctuations in seasonal load). So this leaves us with the daily, hourly, and minute by minute side of the scale. I’ve provided sources elsewhere showing that 10h storage (here) is sufficient for systems with high renewables (to 70%) to assure sufficient levels of reliability and meet LOLE targets for loss of load of 8 hours in 10 years (a standard measure used in power system operation and planning). Two more sources may help to frame the issue: the economic benefits of storage (especially for intervals less than 5 minutes), and national resource estimates from experts in energy planning and power systems design (who recommend 10 – 100 GW of storage for the US over the next 10 years). This appears to be the scope of the challenge that we face in the forseeable future. IT would be great if we could focus on the best way to meet this challenge in this thread (economic impacts, alternatives, technological limits, scalability, readiness of new technologies in the lab, all the issues identified by Barry in the lead article), rather than dithering over faulty assumptions, exaggerated claims, very basic misunderstandings of renewables and power system operations, and sources that nobody takes seriously. So unless anybody else has credible (and fully researched) sources to the contrary, here is how I understand the major dimensions of the problem:
Demand load: there are many seasonal load variables that are important to consider for specific locations in planning for a robust grid, adequate capacity, and energy storage. We are mainly concerned here with daily, hourly, and smaller time periods.
Generation mix: modern energy system utilize baseload, intermediate, and peak resources. How might energy storage change the picture for this resource mix, and any other tools we may use to better match electricity supply and demand.
Time scale: economics of anything beyond 10h storage are not attractive or helpful to making good use of available resources, so we’re pretty much stuck with this time scale. Anything in the 4 day to 7 day range for storing the entire grid is a red herring.
Capacity range: for a system as large as the US, it appears we are looking at 10 – 100 GW of storage capacity added to the grid over the next 10 years.
There is nothing in this picture that looks particularly challenging to me (from a technological point of view), and the solutions available to us will only improve over time. Above, I looked at commercially available NaS batteries and pumped hydro to meet this need. For the NaS battery option currently being sold by NKG for daily load shifting, it looks like an investment of $175 billion over 10 years will get us to our goal (and yield a potential economic benefit saving $625 billion in industrial, commercial, and residential losses from outages). For the pumped hydro option, using the current storage plant in Ludington as an example, it looks like $225 billion will get us to our goal.
On the experimental side of the NaS option (which is a very good battery for load shifting), let’s see if we can improve on the cost and environmental footprint of the conventional NaS battery developed by NKG, which is a modular design based on hundreds and thousands of individual cells. This is not a particularly good use of space, and also has very high material and O&M costs. Prof. Sadoway at MIT has a different approach, and is getting a lot of attention for his single cell reversible ambipolar electrolysis battery, or liquid NaS battery. LMBC is working on a commercial prototype (with funding from DOE, Bill Gates, and Total). Instead of an aggregate of thousands of individual cells (assembled on a modular basis), it’s a “scalable” single cell design with a self-assembling molten anode and cathode metal, and molten sodium sulfide electrolyte (with no separator that degrades over time). While keeping much of the design and materials a secret, the initial prototype will most likely be constructed from molten magnesium (Mg), sodium sulfide (Na2S), molten antimony (Sb-121), some zinc telluride (ZnTe), and a refractory lining (materials that are commonly available, recyclable, and relatively non-toxic). He suggests the size of the battery will be 50 to 100 times smaller than the conventional NHK approach, and have an energy storage cost as low as $50/kWh (with full development). For a detailed description of this battery (it’s design basis and background), I recommend the first half of a 48 minute video on the MIT World website. More here from MIT Tech Review. Where does this stack up with respect to environmental impacts:
60,000 sq meters for 13 GW of load shifting capacity, or 462,000 sq meters for a full 100 GW. No info available on total capital costs, but target storage costs are $50/kWh.
Some here are merciless in focusing on the glass half empty critique, and only seek to look backwards at established technologies. I prefer to see a world full of opportunities (and exciting new development challenges).
Karl, Iceland has little wind and solar. It has geo and hydro – both useful zero carbon energy sources, like nuclear, unlike wind and solar. Iceland is a small country with lots of geothermal and hydro potential nad little electric demand (compared to say the US or continental Europe). Iceland is wonderful, and non-replicably. We need solutions for the world, for 7 billion people, and 10 billion in the future.
What is the efficiency of the quicklime cycle?
Some commenters are neglecting to comply with the BNC Citation Policy, reproduced below for your information:
Citing literature and other sources: appropriate and interesting citations and links within comments are welcomed, but please DO NOT cite material that you have not yourself read, digested and understood. As a general rule, please introduce any and every link or reference with a short description of the material, your judgement on its quality, and the specific reason you are including it (i.e. how it is relevant to the discussion).
@Cyril R.
The efficiency of the quicklime cycle is discussed in detail in the paper I referenced earlier. The authors say that one advantage is that the “Carnot efficiency” will go up with their proposal since it works at 1000 degrees Celsius at the desert site, compared to the around 400 achievable when working with molten salt in concentrated solar power plants.
The proposal also gains efficiency because waste heat can be used in Germany, while it will be just wasted in the desert.
They say their proposal will deliver 11.9 percent of the solar irradiation energy to Germany, as compared to 10.8 percent when generating electricity and delivering it over a 3000 kilometer power line.
For storage purpose the energy density is probably the most interesting value. They give about one third of coal as an estimate.
@EL
I looked at the paper you cited, and I’m pretty sure that they aren’t saying that 10 hours of storage will net you only 8 hours of brown/blackouts per year. Which would be a bold, bold statement given the plentiful time series with multi-day null-generating wind floating around. That being said, I’m not entirely sure what they are claiming. I think that they expect only 8 hours per year where storage (at 30 euros/carbon ton) could find buyers over other generation mechanisms, but doesn’t have any reserves. In short, they are assuming adequate generation and trying to figure out how much thermal generation gets displaced by storage, rather than worrying about whether the generation is adequate in the first place.
I could be wrong… but it isn’t a detailed study of the statistics of wind intermittency (non-Gaussian!), which is what most of us here are worried about.
EL wrote:
Wow, you’re claiming that statements such as gravitational accelleration is about 10 m/s “have no basis in science”? And that the numbers by Uvdiv are wrong without providing a detailed authoritative source yourself?
I believe I’m quite done talking to you, EL. You’re not taking any reference seriously and trash them with bold statements without providing credible references yourself. The ones you cite don’t support your optimism.
You’re one of those “impress with many references and HTML” kind of people, without having read your own or others references.
I’m quite sure this is against site policy, EL.
MODERATOR
Thank you Cyril. It is indeed against site policy but hard to pin down without meticulously checking all references which is an impossible task. Please notify me of individual instances which you may come across and I will take action.
@ Karl. No one has ever made CSP with 1000 degrees Celcius. Even 500 degrees Celcius is proving too much to handle. Making receivers at higher temperatures is not only challenging, it is also extremely lossy, Boltzman-Stefan’s radiative heat losses rising to the fourth power of the absolute temperature difference…
No one has ever made even a power cycle that operates at 1000 degrees Celcius. Currently the best we’ve got is 600 degrees Celcius (supercritical steam) and we have good prospects for 700 degrees Celcius in one or two decades (superduper steam and Helium Brayton cycles). We have open power cycles (gas turbines) that operate at or above this temperature but this is very different technology (combustion) than non-combustion technology.
Even with those developments the best hoped for efficiency is 50%. This is a very poor cycle efficiency; you need twice as much solar to make up for the losses. If you get that both ways you’re down to 25% cycle efficiency, and you need 4x as much solar.
This is clearly not affordable or practical.
You are correct, they run the scale of variable generation up to 68%, and find that storage costs have the potential to be economical (i.e., competitive with CCGT) with a € 30/ton carbon cost in the mix and a reliability performance measure matching LOLE targets of 8h in 10 years (a standard measure used in power system operation and planning). What this means is that storage can replace fossil fuel reserve capacity (on an economic basis) at current costs for pumped hydro (+ carbon costs) and up to 68% variable generation (and still be able to deliver electricity at a performance standard consistent with what we are used to today for their modeled system). They cite Doherty and O’Malley (2005), Ensslin et. al. (2009), and Hasche et. al. (2011) for their wind modeling reference points.
I realize that this is an emerging area of study, and we can anticipate new findings as storage alternatives get more attractive from an economic vantage point, regulatory hurdles are removed, and new technologies start moving out of the prototype phase and into deployment and commercialization. If you have any detailed and substantive research to provide on this front (how much storage is needed per share of variable generation), I’m all ears and would enjoy looking at it. I haven’t done a thorough review of the literature, but what I have seen conforms pretty well with my current understanding. Time scale is important (particularly with respect to economics), but even more important is share of installed capacity for storage, and amount of variable generation in resource mix. Since we have few storage alternatives longer than 10h, few studies look beyond this time scale, and yet many are successful in modeling for energy systems with high degrees of renewables (here, here, and I could go on … which would likely rise the ire of moderators on BNC).
From a technical standpoint, none of this is too complicated (and can be done with current technology). From an economic standpoint, the bar is set pretty low trying to match the price and performance target set by fossil fuels. I’ve indicated where I think some of the benchmarks are located, and some of the price and environmental considerations for getting there. I do admit, matching the price performance targets of “fuel tanks” (as you put it above) are not going to be easy. I think the picture changes quite dramatically with a battery that can be run on the scale of an aluminum smelter (at some 5 GWe capacity per city block), a projected $50/kWh storage cost, and with materials as common and recyclable as dirt (as Prof. Sadoway likes to put it). Until that day, we’re stuck working with commercially available NaS, CAES, PSH, flow batteries, lead-acid, lithium-ion, flywheels, double layer capacitors, and numerous other technologies at bench and pilot scales (and a great deal of heavy lifting from the grid, inefficient fossil fuel capacity reserves, reliable baseload, and demand response).
Sure enough, energy storage, a mix of generation technologies (renewable and otherwise), and intelligent load management are integral to Germany’s future renewable energy targets as proposed for 2050. I don’t see where the the blogger “uvdiv” has accounted for operation of capacity reserves (renewable or otherwise), correlation with demand curve, peak shaving potential with storage, curtailment of wind (when storage capacity is not available), forecast errors, or anything else used in modern power systems operation and management to come up with his result. While it may be a good visual illustration of the variability of variable energy resources, intended to “wow” the casual reader with the appearance of lines squiggling all over the place, I don’t consider his or her “aggregate” representation of the data from “four major utilities” to have any serious thought behind it. It remains to be seen how this energy is being used, to what extent “flexible” generation is an asset or a deficit, under what conditions, and how this picture changes when energy storage is thrown into the mix.
If you’re still looking for my own credible references to the contrary (drawn almost exclusively from the peer reviewed literature), you’re not reading my posts. I’ve made one effort to look at and quantify the problem (in terms of infrastructure need for storage, rising penetrations of renewables in the US, and available technologies to meet this need). If you think it is technically impossible to operate an electricity grid with high renewables (40 – 60%), stable baseload, a modest degree of inefficient fossil fuel peak capacity reserves, and 10h low carbon energy storage at your disposal (at some share of installed capacity), please point me to the research that shows me this can’t be done (because I am pretty sure it doesn’t exist). I believe such a system would yield significant global carbon savings at a reasonable price, and be scalable nearly everywhere in the world (regardless of political conditions, local resource availability, human resource expertise, or waste storage capacity). And it may even help with rising rates of over-consumption and non-renewable resource utilization (driven by low cost carbon-emitting fuel sources). I’m willing to concede storage needs in the US (to take one example where we have a planning document) may be greater than 100 GW over 10 years, and longer than 10h in some locations (where the seasonal variations are the greatest). But to me, this sounds like a pretty fair place to start. And I also concede a better battery (something along the lines of an aluminum smelter in reverse) will pretty much guarantee a shift of development activity along these lines. Since I know you are a strong advocate for nuclear, you’ll also note I don’t leave reliable baseload generation out of the picture.
@Cyril R.
The fact that there is no concentrated solar power electricity generation over 400 degrees is actually the point here. A solar kiln to make quicklime operates at 1000 degrees, which beats the efficiency of making electricity. If people were able to build concentrated solar power electricity plants working at 1000 degrees, that relative advantage of the quicklime system would disappear.
The German government “experience report” of May this year has a chapter on storage which makes a couple of relevant points.
http://k-lenz.de/1009 (Bundesumweltministerium)
First off, they note that storage is not only necessary to assure stability of supply when there is less available. It is also necessary to avoid throttling generation when there is not enough demand to meet supply, a situation that will occur much more often with more renewable capacity.
I comment that this is also true for France with their nuclear fleet. They should use storage instead of load balancing. Run the plants at full capacity all the time and use excess energy to make some fuel or other.
The government report’s estimate for necessary storage in 2050 is between 10 and 30 TWh. That is not possible with pumped hydro in Germany (they say it might be possible in Norway). Therefore, Germany will use gas (wind to gas).
For that reason, that is the main topic of discussion of that report. Some of the recommendations have been enacted in the law reforming the feed-in tariff adopted on June 30.
Once you have wind gas, probably the most efficient way to use it is in 19 kW small power plants built in the basement of buildings, as Volkswagen and Lichtblick are doing right now. They get 90% efficiency by cogeneration and startup times of less than a minute. I have blogged about that yesterday:
http://k-lenz.de/1008
@EL,
If you think it is technically impossible to operate an electricity grid with high renewables (40 – 60%), stable baseload, a modest degree of inefficient fossil fuel peak capacity reserves, and 10h low carbon energy storage at your disposal (at some share of installed capacity), please point me to the research that shows me this can’t be done
When I was in university 30+ years ago we designed a 100 MPG car. There is no research that shows a 100 MPG is technically impossible. All you need is a ceramic internal combustion engine and some carbon fiber composites.
In 1985 Isuzu announced it would have a production ceramic engine by 1990
http://www.chron.com/CDA/archives/archive.mpl/1985_46146/ceramic-engine-for-isuzu-car.html
Here is the 1988 status of the Isuzu ceramic engine
http://papers.sae.org/880011/
And the year 2000 status
http://www.sof.or.jp/en/activities/pdf/06_07.pdf
Here is a youtube video an actual working prototype
There is no research that shows a ceramic engine is impossible.
A working prototype was been demonstrated 20+ years ago.
There also isn’t a production ceramic engine despite decades of ‘promising’ R&D.
There is a big difference between what you can manufacture under laboratory conditions and what can be manufactured under real world ‘mass production’ conditions.
Here is an interesting idea by a German, Eduard Heindl.
http://www.authorstream.com/Presentation/heindl-958931-hydraulic-energy-store-system/
It is a huge rock cylinder, much like pumped hydro under pressure or pressurized air storage with water as working medium.
He claims 1/100th the space, 1/4th of water usage, 1/10th the cost compared to pumped hydro.
It`s circulated widely at the moment in Germany. Some of my engineers have brought it to my attention some weeks ago. It looks like he is forming a team and a group of companys around the idea. The physics seems to be very sound. The pumps/generator might pose a problem. I will ask Heindl about that.
Heindl has his own (German) blog about the topic onhand.
He discusses cavern, pumped hydro, redux flow batteries and H2 energy storage options. His conclusion so far is that only hydro and pressurized air storage can provide the needed storage capacity today. He is citing a lot of German studies on the topic.
forgott the link
http://energiespeicher.blogspot.com/
Jan Mrosik — Others are working on undergroudn pumped hydro (UPH) schemes, for example
http://gigaom.com/cleantech/a-new-energy-storage-option-gravity-power/?utm_source=feedburner&utm_medium=feed&utm_campaign=Feed%3A+earth2tech+(GigaOM%3A+Cleantech)
which is all very well until one starts putiing some prices on such schemes:
http://www.dotyenergy.com/PDFs/Doty-90377-Storage-ASME-ES10.pdf
Basically, at current price ranges, UPS can make its way in the diurnal power market provided there is an assured supply of low cost energy overnight and an assured market for high priced energy during the daytime. This will also be true for the cylinder schemes as well, but possibly the differential between the nighttime and daytime prices might not have to be as great.
Such schemes are most unlikely to be able to offer vast reserves of available energy unless, by some luck of geography, both the upper and lower reservoirs are exceptional.
Sorry I am a bit late into this thread but I thought you might like to see a table that will be in my new book (The Power Makers’ Challenge) which will be out in June next year. [Yes, I admit it is a flagrant plug for my new book but that’s what us poor authors have to do these days.]
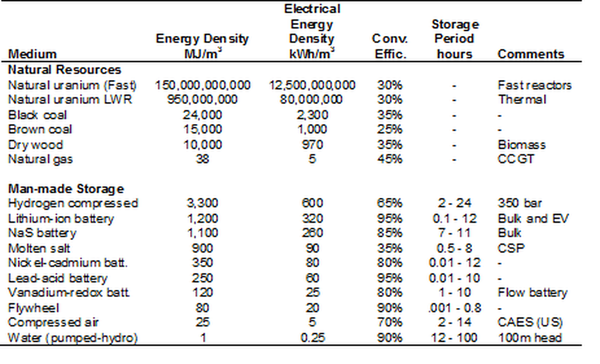
This table has been drawn from many sources and shows properties of varies energy media including natural resources and man made storage systems. You might find the information useful.
I will try to add it as an image but if that fails you can download it from my website.
http://www.energyinachangingclimate.info/storage%20properties.pdf
Martin Nicholson — Thanks for sharing. By the way pumped hydro only rarely can acheive 90% efficiency. More typical is 80%.
@Jan Mrosik
Thank you for that interesting link. I just checked out some of the material. He claims to be able to deliver 1.7 TWh of storage with a VERY BIG ROCK 500 meters in diameter and 1000 meters deep, which would be a very conveniently small sized installation with quite the punching power.
While we’re at sharing links to new concepts, Bill Gates just posted at his website a patented scheme to store energy by moving objects uphill:
http://www.thegatesnotes.com/Topics/Energy/Taking-Energy-Storage-to-a-Higher-Level
That description is rather vague. I could not understand how they plan to reclaim the energy or what kind of setup they have in mind.
I also rather strongly disagree with the idea of someone patenting gravity.
Martin Nicholson, Thank you for that information. I’d like to know how to post tables lihe you have done here.
I would like to see average cost data added to your list. The thing I would most like to know is the $/kW and $/kWh of storage capacity.
I wonder why you say the storage time PHES is 100 h? Is that definded by economic considerations that if you don’t use it sufficiently it can’t pay the capital cost of the plant? If not, why is there a limit of 100 h for storage of water?
@Jan Mrosik and Karl-Friedrich Lenz
The “solid rock cylinder” jacking system for energy storage has been discussed previously on BNC. The people proposing it have clearly never discussed their proposal with anyone who knows anything about rock engineering.
Some questions you might to ask are:
1. How would excavate it?
2. How would you hold the cylinder together in one sold block? How will you stop it collapsing into a pile of rubble?
3. Are you aware the not only will the rock in the cylinder and in the walls of the excavation collapse into a pile of rubble, but if it could be kept intact somehow, the gap will close due to the stress relief – unless you are planning on cutting a wide slot.
4. If you are intending to excavate a wise slot, how will you do it/
5. Have you had a mining engineer calculate the cost of the excavation and of whatever means you are going to try to use to contain it as a solid rock cylinder?
@Peter Lang
Would you have a reference at hand for the previous discussion? Thanks in advance.
Just to be clear, I have no idea if this idea (or that Bill Gates just posted) has any merit. The increases in storage per area of two orders of magnitude are interesting at first glance.
We don’t really need huge numbers like this … we have a grid do we not? We have capacity planning for seasonal variation. I don’t really see the need to stretch the intermediate and peak side of the scale to fit into the baseload box (and build such a thing like we were running our electricity grid in full peak mode 24/7 and 365 days a year). Xcel has some performance data on their pilot NaS and wind turbine integration project. The conclusion: “the technology works.” They see a range of 5:1 – 10:1 for battery capacity scale, in order to:
– Effectively shift wind energy from off-peak to on-peak availability
– Reduce the need to compensate for the variability and limited predictability of wind generation resources
– Support the transmission grid system by providing voltage support, which contributes to system reliability
– Support regional electricity market by responding to real-time imbalances between generation and load
Detailed report here. At 10:1, this amounts to about 100GW of storage for a place like the US (which I have already suggested is a reasonable target, or a high target according to one planning document).
Anybody following developments in isothermal compressed-air storage (for above ground, deploy anywhere, storage tanks in the 4 MW range)? These do not require natural gas to run, but utilize pistons that work at a much broader pressure range than turbines. SustainX is one developer in the States (with DOE funding), and touts a 7x price reduction in storage costs “compared to classical CAES,” 30 year operating life, modular design for scaling to a variety of applications, no geological constraints, and more. Air products is another company breaking away from geological constraints with their cryogenic liquid air (LAES) system for energy storage in 100MW range, 12 hour discharge time, modular configuration, 75-85% efficiency, and more. Adsorption-enhanced compressed air storage is another advanced CAES concept.
Thanks Peter for giving me an opportunity to post yet another table from my new book on energy storage costs. The information is from the Electricity Storage Association and gives cost ranges. The source site is http://electricitystorage.org/ESA/technologies/
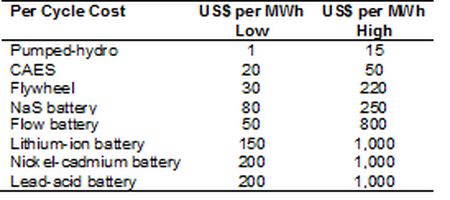
The storage periods shown on the previous table are indicative only to give readers an idea of possible length of usage between recharge cycles. Clearly it depends on the size of the store and discharge rate – so there are no defined limits – only practical ones.
Best not to leave blanks in URL names – try this:
http://energyinachangingclimate.info/storagecost.jpg
Thanks Martin. Interesting figures. It’s nice to have it summarised.
Your list makes it very clear: pumped hydro energy storage is by far the least cost way to store large amounts of energy. Even this method of storing energy is expensive. It is not well suited for unreliables and is best used when matched with plants that generate low cost electricity for baseload.
Of timely relevance to this thread is a new review paper out in the latest issue of Science:
Their conclusion: use of batteries will be dominated by high-financial-yield and specialist situations for some time to come. Forget about massive-scale backup for unreliables.
@EL
“We don’t really need huge numbers like this … we have a grid do we not?”
This might be precisely the problem you seem to be having. Yes. We do have a grid. A massively CO2 emitting grid. If you assume that renewables are a perturbation on the existing grid, so that you don’t have to worry about the existence of a grid, then you are assuming massive CO2 emissions. At which point renewables are a way to spend money for no purpose.
If you want nonperturbative levels of renewables, then you have to show that you still *have* a grid. Any study that doesn’t accept this basic fact is either a fossil fuel plant, or clueless and useless. Worse, renewables, with CFs of 30% and down produce a lot of their power while operating near nameplate capacity. So a 30% renewable contribution means near 90% spikes: 30% renewables (woefully inadequate) is so far from perturbative that it is laughable.
Karl wrote:
Karl, I don’t understand this statement. My point is, receivers operating at 1000 degrees Celcius are science fiction at the moment. So how can you suggest to operate the receiver at this temperature?
@Cyril R.
That is not my suggestion, but that of the authors of the paper I cited. I am sorry to say that I have no access to it right now, since I won’t get back to my university office until Monday. Neither can I access this one:
Multitube Rotary Kiln for the Industrial Solar Production of Lime
http://k-lenz.de/1011
I’m not sure where you get this from the article, it’s too bad it is behind a paywall. The article is timely because it looks to profile the changing landscape of energy production globally, and the contributions of EES (the ‘‘Holy Grail” of the electric utility industry‘) to this emerging picture (10% in Europe and 15% in Japan). They specifically note the US is lagging with a very low 2.5%, and mostly “limited to pumped hydroelectric storage.”
A few quotes:
The article appears to be written to create the impression that people are gearing up for this technology to be delivered on a much broader scale, and for further developments on durability, reliability, long life, and round-trip efficiency to be anticipated in the short run from available technologies (namely VRB or Zn-Br flow batteries, lithium ion, and Na/S). They look at several commercially available technologies, and cite costs as the main limiting factor: “it is not surprising that cost is given as the reason that energy storage is not widely used on the grid.”
I agree with Peter Lang (and the authors of the above Science article) that costs are a central driver. Storage costs need to come down to $50/kWh for EES to become a major component of our electricity system (and to displace fossil fuel capacity when matched with renewables on a cost effective, reliable, and scalable basis). These targets appear to be fairly conservative, consistent, and agreed upon by researchers in the field (nobody has demonstrated otherwise to me in this thread), and at some point we’re going to be getting an assist from rising energy prices for fossil fuel generation (from retirement of old plants, high cost of new construction, non-renewable resource limits, and perhaps even carbon prices). The trend lines seem pretty clear to me at this point, and I’ve suggested one technology (a battery that is optimized for large currents, scalable in GWe range, and low cost) that promises to beat anything out there (and to have very sound engineering principles behind it). We have dozens of very promising technologies at this point (advanced CAES, PSH, flow batteries, lithium-ion, NaS, flywheels, ultra capacitors, metal air), many of them at commercial scales, I’m not sure what anybody is still waiting for (if indeed, there is anybody left still waiting)? And looking at recent development trends in the newly released IEA World Energy Outlook, I’d say people are starting to get the message.
MODERATOR
EL – please note that BNC frowns on the practice of cutting and pasting large slabs of text, even when behind a paywall. In future such instances please give your precis of the contents and your comments on them.
EPRI study from 2010 (“Electricity Energy Storage Technology Options: A White Paper Primer On Applications, Costs, and Benefits“) has a much fuller list (page xxiii – xxiv).
Technologies are summarized by energy scale (MW or KW), application (bulk storage, renewables integration, frequency regulation, transmission and distribution grid support, commercial and industrial, distributed energy storage systems, residential storage systems), maturity (mature, demo, commercial, R&D), capacity (MWh), power (MW), duration (hrs), % efficiency (total cycles), total cost ($/kW), cost ($/kW-h). This appears to be one main citation sources for the Science article above (since many of data points on installed capacity and current technology mix come from it).
How about H2?
Dan Nocera is telling people that distributed power +hydrogen storage will be the way to go.
http://poptech.org/popcasts/dan_nocera_personalized_energy
It seems plausible that we can`t built enough centralized generation anyways so why not save the money we would need to spend on the grid and get it done distributed?
Forget hydrogen, except for niches.
It takes 40% of the energy of a kilo of liquified H2 to compress it.
The AC to AC cycle is 20% to 25% efficient.
I have been involved in 3 H2 fires and their aftermath – it is a difficult material to handle safely. If the general public was entrusted with this job, safety would be an issue.
A very good review of the practicality of the H2 economy is at http://www.efcf.com/reports/E21.pdf, which I found by digging into the Wikipedia references on this subject.
The Wiki article on energy storage is surprisingly high quality and covers much of the material on this thread, although thus far, I have only skimmed it. Don’t blame me if there are errors which I have not spotted – typical wiki – suspend belief until you have checked the references. http://en.wikipedia.org/wiki/Grid_energy_storage
@Cyril R.
I have just checked back on the original paper I was referencing. They don’t provide information there on solar kilns, but just some quotations of earlier papers that address that problem.
I would not worry about the efficiency when the process is a cheap photocatalytic one.
You can still use the energy on the spot to create liquid fuels or methane.
Methane should be ideal for storage, we would not need any more storage capacity but can store it in the gas grid.
It just makes sense that we need decentralized storage and and generation when there are no solutions for centralized storage and distribution costs are too high.
The Big Rock financial analysis assumes that unreliable undispatchable kWh’s are available for 2 euro cents per kWh, and reliable dispatchable kWh’s are sold for 7 cents, acknowledging the fact that reliable energy is worth far more than unreliable energy.
What percentage of existing and proposed wind and solar farms would be economic at 2 cents per kWh?
There was a conference on using gas to store excess wind generation in Berlin yesterday. An article in the Welt newspaper (in German) said that the German existing gas infrastructure would be enough to store 106 TWh, about one sixth of yearly consumption.
http://k.lenz.name/LB/?p=5367
That is more than the German government thinks is needed with 80 percent renewable in 2050 (10 to 30 TWh), figure from the government report referenced in my earlier comment.
I think that grid stabilization is the smaller part of the problem. The bigger part (and the one already happening) is that one has to turn of renewable generation in time slots where there is not enough demand or not enough grid capacity to deal with the electricity.
Shutting down wind parks and solar panels already paid for is an obvious waste of carbon free energy that should be avoided. One other way to do it is to feed the excess into cars, and that is exactly what Audi has started recently.
http://k.lenz.name/LB/?p=5028
KFL I don’t speak German but the clip appears to refer to methane production via the Sabatier reaction. It is oddly reminiscent of another synfuel proposal by a German car maker, namely Sunfuel a type of Fischer Tropsch diesel made from biomass. Thus we have Audi with Solarfuel and Volkswagen with Sunfuel.
I think a viable carbon neutral synfuel must meet 2 criteria
1) energy return EROEI > 8
2) production cost < 10c per MJ thermal.
The first relates to the ‘EROEI cliff’ theory proposed in The Oil Drum that society can’t function without high yield primary energy sources. The second is that the world economy is slowing with liquid fuels at $2/L. Petrol and diesel produce about 35 MJ heating value per litre when burned and I doubt the world transport system could afford fuel costing say even $3.50 per litre equivalent. Methane is ‘hot’ at 40 MJt per kg (ie compressed or not) but we can’t afford any leakage of unburnt gas.
If synthetic methane could meet those criteria the next question is why wind power? Make that wind power when feed-in tariffs have ceased. Why not solar or nuclear? For example you could have temporarily excess nuclear baseload producing synthetic methane to be used in open cycle gas peaking plant. The costs might work out OK if nuclear was very cheap.
You might be interested in another storage technology for 24 hour solar using electrolysis of calcium hydride to produce hydrogen. This technology is being developed by Alternative Designs Solar in the States. I haven’t looked into it, so don’t know much about it. But they claim the process is “over 20 times the energy density of competing storage systems,” and has a very low relative cost because no “pumps or valves are needed for the hydrogen movement.”
They also have a solar gasoline and grid stabilization system that has Arpa-e funding. I keep going back to Arpa-e projects because they have a high level of private sector support and funding … in fact, it’s a requirement for being eligible for the grant. So many companies have already gone past the early development stages, and are working on commercialization and developing markets for their technologies (through demonstration projects, and the like). The acceptance rate for funding among grant applicants is under 1%, so it’s a pretty competitive process on top of it.
@John Newlands
Nuclear power has the same problem (too much supply at certain time slots, leading to wasting carbon free energy by throttling plants). I see no reason to limit any storage to wind, it just happens that early adopters in Germany mainly work with wind parks.
As to costs, that was also a topic of the debate in Berlin yesterday. There are some questions to be resolved there, but I think it is obviously wasteful to shut down already paid for renewable capacity because there is not enough demand.
@EL
Thank you for these links. I have just looked at them. Looks like another interesting idea in the storage area.
I am not sure however if the technology described in the first link adds much compared to molten salt storage, which will also give a thermal solar plant 24 hour production capability and is in use right now. Maybe it is cheaper.
On the other hand, making gasoline is much more valuable. You can move cars, or move the energy from a desert site in form of gasoline (like in the quicklime cycle proposal mentioned above). And it gives another large boost to storage capacities.
@EL:
KF-L is referring to production of methane or similar as a replacement for NG. It would thus be suited for transport and stationary applications, as well as for industrial feedstock.
The H2 process you describe is a closed system – it does not generate H2 for external consumption, eg IC engines. The output is electrical energy, via a Rankine cycle which is going to be no more than 50% efficient.
So, point number one: It is suitable for fixed installations only, to overcome the intermittency of solar thermal power generation. It is, essentially, a battery, used to store and to release heat energy for times when the sun is behind a cloud.
Overall efficiency is not discussed in the article which you referenced. The H2 CaH2 process operates at 800 degrees C or more, so this concept is applicable only to high temp solar collector towers.
The arrangement appears to be for 100% of incoming sunlight to be focussed on the H2 transforming cell, which stores thermal energy as H2 and recovers it by regeneration of CaH2 at a certain temp and P. All of this happens within a range of about 800 to 1100 degrees C and pressures ranging up to say 5 atmospheres.
Surplus heat is bled off from the transforming cell via heat exchange and a single-phase heat transfer gas, to a Rankine generator which is then operated to match electrical demand. So far, so good.
Unused heat results in increased H2 generation and hence increased system pressure. The container volume is constant, so the pressure cycles up and down as energy stored as H2 increases or decreases.
What is not stated:
System capacity in energy and power, although some illustrative power figures were provided.
Size.
Maturity of design.
Poisoning of reactants – does the CaH2 have to be removed, purified and remanufactured and reloaded daily? Weekly? Annually?
What is the overall system efficiency? How much incoming sunlight energy makes it through to the outbound electricity meter after all losses?
Speaking of losses, two which must be overcome are the need to keep the reactor and storage vessels above about 800 degrees C at all times, and heat exchange/generator losses, bearing in mind that this generator will have to operate through a wide range of input heat temperatures.
From a purely thermodynamic point of view, it is interesting that the rate of energy withdrawal will decrease over the operating range of the unit, from 100% at say 1100 C to a low figure at the lower end of the range, perhaps 20% at 800C.
It will be interesting to see how this progresses. Will we ever hear about this notional storage process again, or will it sink like a stone due to energy efficiency and cost?
Nothing you have provided is more than a discussion starter; it fails the “Does it work?” test, because it does not answer any of the practical questions about performance and cost.
You and I have exchanged points of view about emerging technologies previously on this site.
You tend to throw in recommendations which are based on undeveloped proposals which may still be years away from proof-of-concept demonstrations, as this instance.
I express my frustration that, in the world in which I live, only things which have been shown to work are worthy of consideration for immediate use, as is required for addressing pressing problems.
In that, we differ. While I enjoy getting my head around concepts, I cannot base my view of applicability on concepts which have not been demonstrated, preferably at a commercial level.
Level 1. Concept – desk studies, pure research.
Level 2. Proof of concept demonstration.
Level 3. Working demonstration plant.
Level 4. Commercial scale demonstration plant.
Level 5. Design development of first market version.
Level 6. Standardised, refined, repeatable design.
This example you have provided us with is interesting, but is at Level 1.
For me, anything below Level 4 is not yet practical: hypothetical; perhaps interesting, but not fit for service.
We must concentrate, first and foremost, on technologies which are fit for service. All else comes under the banner of RD&D. It isn’t available yet. It does not exist yet.
As I said, I don’t know much about the technology, and am not suggesting it here as a proven replacement for molten salt solar towers (which is sounds to me they are shooting for). Your summary of technology is helpful. Thank you!
Since we’re talking mainly about grid energy and utility scale EES (in MW and GW range), MIT Tech Review has an article yesterday on a new battery electrode design with very high cycle life (40,000 cycles): “In Search of the Ideal Grid Battery“. They turn to prominent researchers in grid energy battery storage for comment: Jay Whitacre (at Aquion Energy), Donald Sadoway (at MIT and LMBC), and Christopher Johnson (at Argonne National Lab). Battery chemistries are inexpensive and abundant (which is key to any real world application), water based electrolyte, electrodes coated in copper-iron nanoparticles on cloth-like carbon substrate. “For grid storage, the battery can be huge,” researchers report, “and using sodium and potassium is very attractive because they are so abundant and cheap.” Efficiency and cycle life are major findings of this research, but not by any means determinative of future development and commercial viability of battery. Whitacre suggests charge capacity is relatively low, Sadoway wants more information on costs, and Johnson wants to see data on round-trip efficiency. Main research article appears here: “Copper hexacyanoferrate battery electrodes with long cycle life and high power” (Nov. 22, 2011).
Batteries have always been a relatively high cost option for energy storage on the grid (when compared to back up fossil fuel generation). As many working in this area have noted, research dollars have historically been nil to scarce and substantive research driving down costs are a relatively new thing (largely the result of recent developments in materials science and nanoparticles, and the search for enabling technologies for renewable energy generation … also a recent phenomenon at a meaningful scale over last 5 to 10 years). It was simply far more practical to start up a peaking plant (than look at low cost energy storage alternatives). “Fit for service,” when most pressing developments in the field are only 3-5 years old, is not a reasonable measure of potential or success.
Karl-Friedrich Lenz, on 17 November 2011 at 9:56 AM said:
“Once you have wind gas, probably the most efficient way to use it is in 19 kW small power plants built in the basement of buildings, as Volkswagen and Lichtblick are doing right now. They get 90% efficiency by cogeneration and startup times of less than a minute. I have blogged about that yesterday:
http://k-lenz.de/1008”
KFL I went to your blog and looked at the links for this VW CHP unit.
In your blog you state:
“Obviously, they will run these gas turbines when the market conditions are best. These small units can be fired up in a matter of seconds, much faster than any other asset on the grid.”
Being an old gas turbine guy this got my interest. However,
After a little research it seems that these units use an internal combustion piston engine and not gas turbines.
Interesting concept though I can’t quite see every household with a VW piston engine in their basement……..but who knows. The Germans are excellent engineers. It should be interesting to see how they engineer their way out of the situation they have brought upon themselves by shutting down their NPPs.
Thx,
GSB
CHP using turbines is not efficient for low temp heat. Small turbines are extremely inefficient. If you want low temperature heat, you’re better off using heat pumps and drive them using electricity, generated in larger, much more efficient combined cycle gas turbines. You actually end up using less gas this way. Moreover it allows more flexibility (you don’t have to burn fuel in the turbine, the electricity could come from other sources…)
Recommended reading on this issue is David Mackay, “sustainable energy – without the hot air”, chapter 21, combined heat and power, p.145-154.
http://www.inference.phy.cam.ac.uk/withouthotair/c21/page_145.shtml
Thx for the link Cyril it is fairly entertaining!!
PS I did not mean to imply that a GT would be good for CHP.
GSB
[…] 24 November: As helpfully pointed out in this comment at the Brave New Climate blog, these are not “turbines”. Therefore I have replaced “gas turbines” with […]
@GeorgeS
Thank you for pointing out my mistake, I have just replaced “gas turbine” with “gas power plant” in my post and linked back to your comment in the update explaining that edit.
@Cyril R.
Thank you for that link.
McKay does not seem to be impressed with this idea. Some of his points are addressed by the German concept. McKay writes:
“in a typical house, much of the electricity demand comes in relatively brief spikes, bearing little relation to heating demand. A final problem with some micro-CHP systems is that when they have excess
electricity to share, they may do a poor job of delivering power to the
network.”
The German system comes with a storage unit for heat solving the first problem and it does an excellent job delivering, because Lichtblick will activate their plants always in the highest priced time slots.
It may be possible to get more efficiency with heat pumps. Discussing that point would seem to lead somewhat away from the storage topic. The main point here is that making gas from otherwise wasted carbon free electricity from any source (including nuclear) gives some massive storage capacity.
Cyril R., on 24 November 2011 at 4:16 AM said:
“Recommended reading on this issue is David Mackay, “sustainable energy – without the hot air”, chapter 21, combined heat and power, p.145-154.
http://www.inference.phy.cam.ac.uk/withouthotair/c21/page_145.shtml”
I read the good parts. The climate change part was good beginners reading. In it he recommends some books on climate change so I downloaded “The Science and Politics of Global Climate Change”,Dessler.
GSB
Karl-Friedrich Lenz, on 24 November 2011 at 10:43 AM said:
“The main point here is that making gas from otherwise wasted carbon free electricity from any source (including nuclear) gives some massive storage capacity.”
Wouldn’t it be more efficient to just store the excess energy in batteries?? What is the justification for making gas over storing it in batteries?? I think the conversion efficiency comparison is something like 50% for making gas and 90% for putting it in batteries. An EV would be the perfect place to off load the electricity and then displace the oil that would have been required for transportation.
The first occurrence in this comment string of the ambiguous phrase — in North American english, anyway — “making gas” comes shortly after KFL’s reference to making gasoline, so I’ll assume it means that.
GeorgeS, for a lower energy conversion efficiency, you get something. In fact, you get a lot: you get fuel that works in cars that people who voluntarily buy cars voluntarily buy. Efficiency is no good if it means efficiently staying in labs and on magazine covers. To the nearest hundredth of a percent, the fraction of car purchases that are pure EVs is, correct me if I’m wrong, 0.01-0.05 percent.
If it’s actually less than 0.005 percent, and so correctly rounds, if two places are being given, to 0.00, don’t hesitate to correct me in that direction.
G.R.L. Cowan, hydrogen-energy fan until ~1996, on 26 November 2011 at 3:15 AM said:
“If it’s actually less than 0.005 percent, and so correctly rounds, if two places are being given, to 0.00, don’t hesitate to correct me in that direction.”
Actually the last time I checked the current production rates for the Nissan Leaf and the Chevy Volt were around 10000/ month. At least it’s a start.
10000X 20 kwh=2 GWh/mo of storage capacity.
Batteries and Chevy Volts:
George S’s 2GWh/month of storage capacity isn’t all going to be available for storage.
Some will not be plugged in – on the road, parked, etc.
Some will already be fully or partially charged.
Even a bold assumption would be 25% of 2 = 0.5 GWh/mo.
It makes little difference whether these batteries are stored in a shed in Alaska or in hundreds of millions of Volts – to do the job of serious energy storage for a wind and solar powered economy, the combined total mass of batteries will be many tens of millions of tonnes, much of which will be metals such as lithium.
As was pointed out in the introduction to this thread, the logistics are unsolveably challenging and the world’s resources of lithium and some other candidate metals are finite.
The nett outcome of all of this hypothetical storage is what? No more energy will be made. This whole exercise is planned so that valueless energy which is made when it is not needed is supplied when folks are relying on it, after a heap of effort and cost and losses and resource utilization.
It appears to me that this unmanageably large problem is just adding to a problem of our own making – the need to convert unschedulable energy into a controlled energy stream, fit for the market, when other, reliable energy sources are available.
Even the most basic root cause analysis would indicate that the problem should be addressed at its source – that something has gone badly wrong at the generation end of things. Trying to fiddle with the means of delivery and/or the demand aspects of the situation seems to me to be doomed because we are forcing more inefficiencies into the system whilst concurrently meeting less of the consumers’ wants and needs – ie, decreasing satisfaction.
Basic market theory indicates that less satisfaction or higher costs will always result in lower sales.
The question becomes:
“What combinations of batteries (or energy storage) are available that, when coupled with intermittent energy sources such as wind and PV, will better satisfy all or part of the energy market?”
By phrasing the question in this manner, the role of the market is recognised. It throws into sharp focus the need for any successful system to satisfy perceived market demands which themselves arise from consumers’ wants and needs.
Storage is not the problem and it is not the answer. The market generally does not gain satisfaction from storage per se, except for transport applications.
Ultimately, it is likely to be futile to try to harness the storage benefits which transport applications seek in order to re-shape the supply chain for a much larger market, stationary energy demand. Even the slightest increase in cost or decrease in satisfaction for transport uses will come at a high price to the supplier through loss of market share.
Another way of saying this is to recognise that the more lead a horse carries in a race, the less likely it is to win. Put sufficient lead (or lead-acid battery) into the horse’s saddlebags and it will always lose.
The day when wind and solar proponents bring to market fit-for-purpose products will be the day that these same firms include batteries or other forms of storage in their own prices. Until that day arrives, I am sure that wind and solar will remain playthings of the rich or suppliers to niche markets. For the wider markets, their product is not yet fit for purpose.
Australia’s Trade Practices Act would, if not for politically inspired distortions of the marketplace via a range of public and privatised subsidies, have prevented wind and solar in their present forms from being sold in Australia. See, for example, Sections 52, 53 and 71 of that Act.
Our energy problems are NOT primarily storage. The perception that storage is a solution thus fails to recognise and to deal with the real problem.
To the extent that storage IS a problem, it is a problem only for (a) the transport industry and (b) the manufacturers of unreliable energy sources. It’s up to the manufacturers of transport and of unreliable energy sources to provide storage as part of their product, not as optional extras.
@GeorgeS
I don’t have any preference. Batteries will work just fine for some situations. As noted in this thread, changing all vehicles to electric gives some serious storage power.
Charles Brush used a network of batteries in his basement to power his mansion from wind only in 1887 (source: Daniel Yergin, The Quest, page 592), which makes him probably the first person to go 100% wind for his own consumption in history.
However, as the German government report of May 2011 I referenced above noted, batteries won’t work very well to store excess energy from wind farms at the location of generation. You would need very large and expensive batteries for that.
Link to German government report repeated for convenience:
http://k-lenz.de/1009 (Bundesumweltministerium)
JB, on 26 November 2011 at 9:03 AM
Well stated JB. Your remarks about the role of markets generally get lost in the blind optimism of technology.
We can build layer upon layer of complexity to “solve” the “problem” of intermittency (storage, smart grids, EV’s, TOU pricing, smart appliances, changing behaviour, combining different intermittent technologies, geographic diversity, carbon pricing, etc..), OR we can just bypass the intermittent generation and build dispatchable, reliable, low-emission generation…..
Karl Friedrich Lenz wrote:
And as pointed out to you, this is much too expensive if used as true storage (charge-discharge) due to the high performance nature of car batteries. The only realistic scheme is one where you use flexible charging to schedule things out a bit, this gets you about half a day of ‘equivalent’ storage capacity. This happens to be a great match for nuclear since most of the mismatch in a nuclear grid is the nighttime/offpeak excess, this can then be reliably used to charge commuter electric/plugin hybrid cars.
With wind, you have need for much more than half a day of storage – in fact 5 days wouldn’t be enough. Storage on this scale is both environmentally damaging/resource hungry and way too expensive, so what we’ll get is lots of fossil ‘backup’ burning.
Actually the word ‘backup’ looks to be the energy understatement of the century, since this would be a mostly fossil grid with some wind and solar that hops along whenever it is available (hint: this is not available 80-90% of the time, guess how much fossil you need to use).
@Cyril R.
Yes, I read that from Graham Palmer and from you, to which I answered that McKay does not discuss how the market will play out for that particular option. You may be right that this will prove to be expensive. That doesn’t change the fact that this is one option which might be appropriate for some situations.
I won’t discuss the merit of nuclear in comparison to other options here. This thread is about storage, which is useful whatever mix of generation methods you prefer.
A nuclear grid needs far less storage than a wind+solar grid, at least one order of magnitude for the same reliability level. I believe this is very much relevant to the discussion of energy storage. I also believe, based on previous discussions with you, that we are both interested in getting off of fossil fuels and want a plan that adds up, like Mackay. This means no 20% wind and solar, 80% natural gas grids, as most people today are looking at. It means a 90% zero carbon grid. Once you start looking at that and compare real wind+solar output to real electricity demand patterns, you’ll quickly run into a bunch of granite walls regarding how to match these.
There is a problem of making choices: you can never do just one thing. Install wind turbines, it means for every kWh of wind you’ll need 2 to 4 kWhs of fossil fuel to match things out. It is not a choice of nuclear and unreliables, it is a choice of nuclear only, or unreliables and fossil, or unreliables and nuclear and fossil.
This is true unless you have low cost efficient storage, which brings us back to the quantity of storage required, and its efficiency. The wind to gas is absurdly inefficient which is a showstopper. I’ve never seen any indication that we can build low cost high efficiency energy storage on a sufficiently massive scale to avoid using fossil in a wind+solar grid. Efficient, low cost, scaleable energy storage is what we’ll need, not 2 out of 3. All schemes proposed so far fail in at least one of these three categories.
@Cyril R.
“It is not a choice of nuclear and unreliables, it is a choice of nuclear only, or unreliables and fossil, or unreliables and nuclear and fossil.”
If you can choose nuclear only, it follows as a matter of logic that you can choose nuclear plus renewable.
While it is true that you don’t need storage to stabilize a grid with nuclear capacity, stabilizing is not the only thing storage needs to do. Storage is also necessary for using carbon free electricity that would be wasted in time slots where there is not enough demand. It does not make much sense to throttle the French nuclear plants when they could be producing fuel instead. And storage is necessary to transport energy from the large scale desert projects coming up as long as there are no grids to get the electricity from the Sahara to Germany.
Karl, you’re not thinking this through. Please try to read and understand my entire comment, not just the first sentence so I don’t have to explain everything twice.
Nuclear plants won’t throttle down to accomodate windy and/or sunny conditions occasionally, in a nuclear + unreliables grid. Wind and solar are not capacity sources, because they are not there most of the time and cannot be dispatched when necessary. It is “run if it is there”. The point then, is to displace fossil fuel and its emissions when sun or wind are available. If you already have sufficient nuclear capacity then there is not any point in going for the extra investment of the unreliables. There is no emission to displace. You are displacing one clean energy source with another at great cost. It is marginal and expensive. This money can be spent so much better on less marginal energy projects in the world.
If you have the nuclear plant you will run it as much as you can. The cost of maintaining solar and wind alone, without any capital considerations, is higher than nuclear fuel cost. The cost of transporting electricity from the Sahara to Germany, is higher than the cost of nuclear fuel. If you throttle the nuclear plants the cost of maintenance increase and the fuel wears out faster and unevenly, requiring premature replacement (in terms of kWhs generated per fuel assembly).
What you are claiming is that, if one factory produces goods at three times the cost of one other factory, the other factory will shut down to accomodate selling the more expensive goods in the market. Any child can see, the opposite is happening. The reason why the Germans are building any PV at all is due to massive subsidies, 10 to 20 times the actual value of the PV electricity (the marginal production value, a few cents per kWh worth of fuel and O&M displaced in a coal or gas plant). It is not hard to see that Germany must continue on with such massively distorting subsidies while the price of PV is above a few cents per kWh. Even at that point it is still a dubious energy source.
It gets worse, because most people in Germany use PV as part of an excuse to shut down nuclear plants. These get replaced by coal and gas plants. These must run because the solar and wind is not there most of the time. Essentially locking in Germany in fossil fuel grid for several more decades.
If everyone was like you Karl, the Germans wouldn’t be in such a pickle. Reality is unfortunately much more ideological and much less rational. People think they can displace nuclear with solar and wind. They cannot do this. What they are getting is a fossil fuel nightmare.
Regarding power from the Sahara, I should mention that Desertec, the most ambitious CSP energy plan in this respect, aims to get only 15% of Europe’s electricity from this desert power import, by 2050. Even that is extremely optimistic, requiring various CSP learning curve and energy storage cost reductions. The more recent molten salt storage costs, as discussed above, do not meet the cost estimates of several years ago. In fact they have increased in cost greatly.
By 2050 we need have almost solved the situation. Where is the other 85% going to come from? Remember, you need to meet the condition that the demand is met at all times, so you can’t pile up one unreliable upon another like Mackay does (almost all of his suggested renewables are unreliables). You need to actually look at real grid demand information, and real solar and wind output on a national scale. This is available on the internet, Google it.
Here’s one good reference, from a solar inverter manufacturer (we can’t expect bad results from such a biased source)
http://www.sma.de/en/news-information/pv-electricity-produced-in-germany.html
Current production, at about 15.00 hours, is 9% of peak. That’s essentially nothing, right when German demand starts to climb from people turning on their televisions and lights (it is getting dark already in this corner of the world, at this time of day and year). By 16.00 hours it is expected to produce nothing. Zulch.
What is Germany going to use for the winter when the sun is out with the daisies? Are they going to have special nuclear plants that operate only in winter? Of course not, if you have those you might as well run them in the summer too and forget about the high cost grid destabilizing thermal cycling inducing unreliables.
Here’s what Germany’s demand looks like:
http://energyfromthorium.com/forum/viewtopic.php?f=39&t=2689
Seems to me the the most logical solution from a global warming point of view is to have lots of nuclear power plants charging lots of EV’s at night when NPP’s demand is low. This way you have killed two fossil fuel problems with one stone. ie you have eliminated fossil for electricity production and also for a majority of your transportation.
For larger vehicles where batteries don’t cut it you run extended range electric vehicles that use batteries but are backed up by a range extender that is a hydrogen fuel cell. Make the hydrogen with excess nuclear power at night.
Cyril … do you have a references to substantiate the claim that wind and solar are not capacity sources? Many Independent System Operators (ISOs) calculate capacity credits in range of 5 – 35% for wind (taking into account multiple considerations from different siting characteristics, seasonal production profiles, learning curves, dispersion of wind farms, number of connected plants, isolation of grid, and newer methodologies for correlating wind output to peak demand loads). This thread is also about bulk storage technologies for grid stabilization, peak shaving, load leveling, frequency response, fuel production, and more (with some technologies reporting high round trip efficiencies of 90% or more, 30 year or more cycle life, geological independence, and low storage cost of $60/kWh or less), which is only going to improve over time. We have many locations where renewables are currently approaching 20% of total power output (California is one example), and that is a huge amount of carbon free energy (exceeding nuclear in many locations). And most of this has taken place with no storage or additional capacity thrown into the mix (see California ISO study), but just conventional management approaches (distributed generation, flexible operating reserves, well functioning markets, etc.). When storage is considered, study prepared for California Energy Commission (2010) concludes current policy target of 33% is an “effective” and “reasonable” goal (with costs as the only limiting factor). (Inflammatory remark deleted.)
MODERATOR
This type of remark causes an unproductive tit for tat argument.
[…] now there is a thread about energy storage, in which regular contributor “Cyril R.” posted this: Karl, you’re not thinking this through. Please try to read and understand my entire comment, not […]
@Cyril R.
As I said before, if nuclear only is possible, it follows that you can have nuclear plus renewable, if only storage is of concern, which is the topic of this thread. It might be open to debate (not on topic in this thread) what the relative merits of those solutions are considering cost or whatever other aspects people are interested in.
I just posted a long reply to your comment on my blog, as to avoid drifting off topic in this thread.
As to Desertec, my point was only that storage in chemical form (like the limestone concept mentioned above) is necessary for transporting the energy until you have built the power lines.
On a smaller scale, the wind to gas solution now favored in Germany to get the necessary 10 to 30 TWh of storage will also replace the need for some new power lines. Chemical storage can do space-shifting as well as time-shifting.
EL, re capacity credit of wind, I posted this on the geographical wind smoothing thread:
http://bravenewclimate.com/2011/10/29/gws-sg-es/
AEMO has recently lowered the usual 95% availability for conventional generators to 85% for wind in South Australia, with the resulting firm capacity “lifting” to 5% in summer and 3.5% in winter. Reference to the NEM data shows that adding in the Vic, Tas & NSW doesn’t increase the firm capacity much.
http://www.aemo.com.au/planning/SASDO2011/documents/CHAPTER5.pdf
(The comment to which you refer has been deleted.)
I know, I saw that in the previous thread. This looks like a promising and favorable report to me? You’re getting 20% total electricity consumption from only 14 wind farms (which is a large share of energy by any measure) … and with no storage in use. Some wind plants are operating with capacity factors in range of 35.5% – 41.5% (with learning curves increasing). Fluctuations greater than 50% of total installed capacity are essentially nil, synchronized output fluctuations are “infrequent” (p. 70), wind performs well during periods of low demand (providing additional network development opportunities, p. 75), the “general trend shows variability falling as the number of wind farms increase” (p. 63 and p. 72), South Australia is exporting electricity for the first time (and imports are decreasing), the lower 85% confidence factor should improve reliability during times of peak load (they are looking at better approaches, such as a simulation technique), and much more. Power systems management has become “more challenging” (p. 71), and will likely require additional services from storage, flexible reserves, better wind forecasting models, or something else at higher penetration levels. If I had to pick out a negative, it’s that profits from fossil fuel generators are increasing, and wind energy profits are holding steady. Overall wholesale electricity market prices appear to be dropping with wind power in the mix. What troubles you about this report?
The problem about wind is that when they fail, they tend to fail all at once. It’s the resource not the turbines that are unreliable. Unlike conventional generators, this is common mode failure. With conventional generators, you have a simple statistical solution: build more plants, the plants are statistically seperate from each other so the reliability builds up greatly. With wind you are limited to regional low wind speed times. The report discussed above is largely irrelevant because it does not analyse the real issue, which is how do you power a country with wind if you don’t want to use a lot of fossil fuels.
Here’s a detailed discussion and destruction of the deception put forward by wind advocates:
http://uvdiv.blogspot.com/2010/03/uptime-downtime_07.html
Here’s another great reference that integrates the real time wind output of all of Spain’s wind turbines:
https://demanda.ree.es/eolicaEng.html
Notice as you scroll through the dates, that there is huge intra and inter day, even inter week and month variability.
This is certainly enough reason to be troubled about powering countries with unreliables such as wind if we can’t use a lot of fossil fuels.
Yes, detailed studies (in a peer reviewed setting) show that Spain can reasonably reach 33.6% energy mix from wind on a technical and economic basis (and with no storage in use). The remaining 50% could come from “constant inflexible base load” (perhaps low carbon as well) and 16.4% from gas turbines. With rising demand estimates for the next 4 decades, this 33.6% share of wind (on an economic basis) would amount to some 70.5 TWh of energy, and would be entirely sustainable, carbon free, affordable, and create zero long term waste. I’d say this is a pretty good result, and also might be significantly increased with efficient and low cost energy storage over the next 40 years (replacing gas turbines as a flexible energy resource). Throw in nuclear and 24 hour CSP for baseload, and this looks like a pretty attractive, affordable, sustainable, and low carbon future to me (and with very minimal use of fossil fuels … and none of it from coal).
I don’t consider daily generation profiles for wind to be terribly informative, or the German data presented by “uvdiv” (which are taken entirely out of context and are not correlated with load, as discussed above). There are much better sources than these to make substantive and critical points about the variability of wind, energy storage as an enabling technology, and the trade-offs of different generating technologies with respect to costs, carbon mitigation potential, flexibility, long term waste production, sustainability, fossil fuel lock-in, common mode-failures, and more. I’ve mentioned it above, but it does nobody any good to keep repeating the same overstated and faulty claims from poorly designed and inadequately presented research (especially when I have made every effort to rebut such claims with substantive and peer reviewed research to the contrary). If we hope to avoid the tit for tat exchange, as suggested by the moderator above, I would recommend drawing on different and more relevant research that does not need to be rebutted at the most basic levels of assumptions and research design.
UPDATE
Here is the correct link for the spanish study:
http://dx.doi.org/10.1016/j.enpol.2009.04.012
@EL : So you agree with a scenario where 33% of energy comes from wind, 50% from nuclear and 14% from fossil sources ?
I’m not certain it’s feasible to go as high as 33% for wind, but it’s certainly not useful for pro-nuclear people to try to fight for 0 % of wind or PV.
I have a problem with people who tell me “forget nuclear, it’s outdated, we’ll do wind instead”; but I can accept that someone wants to do some wind, as long as it’s stay in the envelope that’s actually doable, let’s say 15 to 30% of the full capacity (30% does seem a lot, but if we do 15% and find out it’s still materially reasonable to go to 30, why not). The main goal should stay to reduce the ugly dependence on fossils, and it’s quite too idealistic to imagine only one option for that will ever be used by everyone.
Also wind can be a good match for hydrogen/methane generation. The efficiency is bad but it’s bad also if using nuclear as a source, and the need is really there for cars whatever the cost. EV is much more efficient but doesn’t scale because there’s just isn’t enough lithium available on earth (in addition to not being the only things that requires lithium). It’s quite surprisingly cheap and easy to convert a car to LPG/autogas, cost between 2 and 3 000$, already too expensive in a day of cheap oil, but it could perfectly be massively done when oil becomes massively expensive (and could be subsidized).
Liquid Petroleum Gas …
when petroleum, and therefore LPG, becomes massively expensive, yes. Lots of things could perfectly be massively done.
Yes. I have no problem with this. But I see baseload in a much broader sense than nuclear (with geothermal, CSP, and CCS also available as future alternatives). I know of no study that suggests 33% wind (with proper grid support and no storage) is not a feasible option from a technical standpoint. This study suggests that for the Spanish case, the above generation mix (with wind to 33% of total energy output) is also economic. Over the long term, I think we can do much better, particularly with energy storage, offshore turbines, and demand response as part of the picture. I think the technical hurdles we face are far less challenging than the social, economic, or market headwinds that focus our attention away from long term solutions, and blow in the direction of natural gas, oil, and coal (which are enabled by everything from poor building design, inefficient automobiles, wasteful consumption, and a great deal of short term thinking on convenience, deferred costs, sustainability, and industrial development curves). I would like to think we can get ahead of the problem, but this doesn’t seem to be our habit.
For a more aggressive approach to carbon reduction goals in primary energy use (80% below 1990 levels) by 2050, I would take a serious look at the following study (recently published in Science Express): “The Technology Path to Deep Greenhouse Gas Emissions Cuts by 2050: The Pivotal Role of Electricity” (Williams, et. al, 2011). Available on-line (and without purchase). It is a major study from leading authors in the field, including the head of the Climate and Carbon Sciences Program at Berkeley National Labs (Margaret S. Torn). Here are some of the broad outlines:
Study looks at “physically realistic modeling” for deep greenhouse gas cuts in primary energy use (80% below 1990 levels by 2050) for California (“the world’s sixth largest economy and 12th largest emitter of GHGs”). Major finding is that you can not meet this goal without significant gains in energy efficiency and electrification of transportation sectors (decarbonized electricity supply does not get you there alone when compared to other studies). Central to study is “realistic modeling,” which draws on stock-rollover methodology, historic uptake rates, resource constraints, electricity system operability (particularly as concerns intermittency of renewables), coordination of investment, and commercialization of future technologies (anticipated contributions from energy storage, CCS, smart charging of electrical vehicles … either as options, or in remote outflow years). From an interview with author (Physorg): “This study is meant to guide decisions about how to invest in our future. Assuming plausible technological advances, we find that it’s possible for California to achieve deep greenhouse gas reductions by 2050.”
What are their key findings: energy efficiency holds the key (future goals are near impossible without it), and we need to “improve energy efficiency 1.3% per year on average for 40 years.” Smart growth, rooftop PV (10% of electricity demand by 2050), biofuels play a small role. More significant, we need broad electrification of transportation and other sectors (75% replacement of gasoline in transportation, 65% replacement of non-heating/cooling fuel use in buildings, 50% replacement of industrial fuel uses), and decarbonization of electricity supply (90% generation from carbon free sources by 2050). Since it is generation technologies that interest us most here, they don’t come up with a single model to achieve 2050 decarbonization goals, but recommend four models (high renewables, high nuclear, high CCS, or mixed). The suggest 74% as the upper limit for renewables given California’s resource endowment and deployment of enabling technologies: energy storage, forecasting models, fast response peak generation, and shift in load curves from smart charging (p. 3). This will be higher or lower in some areas concerning resource constraints. Most interesting, “Our results show that generation mixes dominated by renewables, nuclear, and CCS, in the absence of cost breakthroughs, would have roughly comparable costs, raising the present average cost of electricity generation by a factor of about two” (p. 4). They also note the overall price tag for technology deployment would be around $1200/person over 40 years, and would be offset by economic savings in health care outcomes, deferral of climate impacts, and cross-sectoral economic impacts.
I’d like to suggest if you’re not reading studies like these (and there are many available at this point), you’re not seriously engaged in practical discussions of our most pressing and significant energy challenges (which isn’t to say less practical thought experiments around more narrow partisan concerns isn’t also of value). But at some point, we have to force ourselves to keep returning to the larger picture (and from many vantage points). This study suggests “the three key energy system transformations identified here [energy efficiency, generation decarbonization, electrification] are broadly generalizable to developed economies” (p. 30). And it’s this issue that concerns me most. I’m an anthropologist who works in rural, low income, and non-western communities (and work on a variety of issues … climate change among them). I have a great many doubts about the practicalities of a “high nuclear” option described in this report, and it’s applicability to many regions of the world which lack a current high degree of political or economic stability to allow a robust regulatory or debt financing environment to emerge (particularly over a 20 to 50 year timeframe). So “sociopolitical considerations such as equity, local initiative, and adaptability” (p. 5), referenced in the report, are also of central concern to me. I’d appreciate any other substantive thoughts on this report. In fact, I recommend it for a comprehensive discussion on this site (and perhaps even it’s own blog post).
This is utter nonsense. Even the pro-renewable CEC (California Energy Commission) report disputes this absurd number. Why? Because it would require a huge investment in non-commercial scale storage that *doesn’t even exist*. It also requires smart charging which is not going to happen until, and IF, battery storage technology gets better (which it’s not, as of now). No one is going to allow the grid to *drain* their car battery. “Range Anxiety” being the second biggest hindrance to EVs.
EL this kind of report *requires* in the State of California a shift of only *3%* of the population that uses public transport to over 50% or more. This is not happening in MY lifetime and I’m 55 years old. It requires that energy storgage capacity of 20GWs…again…there simply are no plans for this *at all*.
The reason this nonsense can get through peer reviews is the math. The math says “if x = n then we can get y = z”. The operative ‘operator’ here is “if”. So of course it can work. Even the silly Jacobson paper got through peer review based on making the math work.
You need EVERY one of those “ifs” to work, or it falls apart.
David
It’s not a planning document (and you shouldn’t evaluate it as such). The main purpose is to “guide decision on how to invest in our future” with an emphasis on plausible technological advances (which are forthcoming in every technology, nuclear included). If you’re solution is to invest in nothing, they have a projection for that too … and it’s 875 Mt CO2e in 2050. If you’re 55 … what did the world look like in 1956, and how similar is that to the world we live in today (you appear to have replaced your pen and paper with a novel and technologically advanced communication device)? Energy storage to 20 GW is entirely relevant and germane to this thread, and is discussed in much detail above (with few technological obstacles standing in the way, but mainly economic ones as has been discussed). What will the cost of gasoline be in 2040 or 2050 … don’t you think this will be a significant driver to the large scale adoption of more cost effective alternatives (even if 100 miles per charge remains the technological limit in 40 years, which is highly unlikely)?
This is not what they propose. Smart charging “of electric vehicles flattens the average daily load curve, reducing capacity requirements” (p. 7).
This discussion about storage options has not uncovered any plausible large scale energy storage options with a competitive or even an affordable price.
It has now veered off-course into grossly optimistic, innumerate and speculative wishful thinking, devoid of actual physical description and costings. The basis for these is that “plausible technological advances” may be of benefit in California in 40 years’ time, that people will just change their ways and everything will work out fine due to annual efficiency improvements, majority use of public transport – electrically powered, and more besides. It’s clear that this probably will not work for California, but worse is yet to come.
Developing nations are going to get by without nuclear power, despite the fact that there are no realistic alternatives, if these nations are ever to have healthy energy budgets.
We read the following paternalistic dismissal of nuclear options for up to half of the world’s population:
Well, that has fixed that, then!
Over the past couple of years, on this site and elsewhere, the nuclear war bogey-man which is at the core of that pronouncement has been repeatedly skewered. See, for instance, the comments from 2009 at http://bravenewclimate.com/2009/09/07/is-our-future-nuclear/. There have been many more in the intervening 26 months. One thing about which I am certain is that nuclear power does not have as a corollary the proliferation of nuclear weaponry, yet here it is again, trotted out as though it is a certainty.
What is certain to me is that any solution to the world’s carbon dioxide emission problems which does not also provide a secure energy future for the less developed nations of the world is not a solution at all.
As an anthropologist, EL presumably he has deep insights into the probable outcomes from denying a real future to half of the population… risks of mass migration, of war, of political turmoil, of overuse of resources eg timber and coal, simply because there is no alternative. These are exactly the types of circumstances which are true corollaries of perpetuating the status quo!
Perhaps one of the ways in which Australia and other lucky nations could meaningfully assist our less fortunate neighbours would be to help them to acquire reliable energy sources, including, where appropriate, nuclear power and modern distribution systems.
The consequences of a non-nuclear-powered Third World, it appears to me, is exactly the same as for a non-nuclear-powered First World – unavoidable climate change.
EL has recommended that a discussion take place on this site regarding this latest piece of published speculative nonsense.
I suggest that a far more worthwhile discussion could cover the means by which to assist Third World countries to transition to zero carbon options, because anything that works there will also work in the First World. We need solutions that do not cost hundreds of billions of German and Spanish and Italian Euros to migrate single digit percentages of these nations’ energy sources to PV, wind and solar thermal.
We need low cost, reliable, zero carbon power and transport solutions which do not come with 80% or even 20% fossil fuelled backup requirement and with commercially and environmentally responsible storage options… which brings us back to storage, the topic of this thread.
As with so many things in life, the cheapest energy storage option may well be to minimise the need for storage at the design stage, yet here we are again, being asked to adopt a Heath Robinson (http://en.wikipedia.org/wiki/W._Heath_Robinson) or Rube Goldberg (http://en.wikipedia.org/wiki/Rube_Goldberg_machine) design for our energy future – a complex assembly of barely “plausible technological advances” when simpler and more reliable solutions are staring us in the face.
The problem is that we are notoriously bad at predicting what the future will hold. In the 1950′s, the future included flying cars and nuclear powered vacuum cleaners. We got nothing of that, but instead we saw the IT revolution (and a lot of other things). Advanced communication devices that were just not imaginable in the 1950′s. Just go back 15 years and think, Spotify in a mobile phone. Several millions of tracks available anywhere, anytime… Mind boggling.
It reminds me of the swedish physicist and Nobel laureate Hannes Alfvén who discouraged sweden to pursue nuclear power because soon fusion power would be available instead. The swedish politicians apparently did not listen too carefully, of which I’m grateful. Sweden has now the largest nuclear power generation per capita and essentially a carbon free electricity production.
We cannot base policy decisions that ultimately concerns our entire welfare on assumptions about what might, should or will probably be available in the future. If (not when) someone comes up with the perfect energy storage, we could just build solar and wind power in masses and charges these, …things. But in planning for the coming decades we need to rely on things that are available and proven today. Any country that has so far tried to pursue renewable power has just locked itself into fossil fuels (think Denmark), and this is not an option for the next 50 years. Thats the reality today, and thats what we have to face.
EL makes wild claims and pretends to be supported by “peer reviewed work” on high wind energy penetrations. Actually when I tried to open his link, this is what I got:
Other than that, EL appears to be what I call a “floater”, floating claims without ever answering any detailed critique against these claims.
EL asks for a correlation of wind output with the electric load. I provided this in the link above to the energyfromthorium forum, where a fairly constant German electric load is seen. This then compares to the wildly swinging German wind power graph from Uvdiv. Can you put two and tow together?
Here’s another one, from Texas:
http://uvdiv.blogspot.com/2011/08/texas-electric-grid-declares-level-1.html
As we can see, wind power is (again) substantially de-correlated from load.
Wind power is just very jerky and unreliable. You can’t run countries off it. To quote an Australian politician “its a pity that you can’t. But you can’t”.
http://uvdiv.blogspot.com/2010/03/uptime-downtime_07.html
This isn’t surprising because of the V^3 power law of wind power with wind speed.
If EL wants to avoid a tit for tat argument line, he will have to supply a detailed numerical rebuttal from the above references, not some dead link to supposed peer reviewed work.
EL wrote
This is a good example of the “floating” behaviour. EL links to a study by Pacala and Socolow, well known to all of us. It is about stabilization wedges, an approach that COMPLETELY omits energy storage and intermittency. Certainly by omitting the intermitency issue altogether and simply adding up the yearly kWhs from solar, wind, and other unreliables, you can make it look like we can solve the problem. Any detailed analysis of real grid demand and real unreliables output, will quickly show that this approach is grossly inadequate and about as unrealistic as it gets.
Back of the envelope calculations are not a good example of peer-reviewed work. I’m surprised that Pacala, Socolow, and other researchers are getting away with publishing such simplistic nonsense. It promotes false optimism about a growing problem, and it makes me wonder about the quality of “peer review”.
Cyril … the broken link was updated in a subsequent post (some 4 minutes after the original post). And with bold letters ” “UPDATE” posted over the top. I take no pleasure in pointing out these oversights on your part.
Yes, I agree with you, and think this is a very important concern. Which is why I am so interested in low tech zero carbon energy sources that can be deployed to scale without large capital, waste storage, and oversight requirements (if just to provide lighting or to power computers as a pre-requisite to raising local educational standards). The World Bank has just such a program for Africa. Diesel is already an option for many remote locations, and is currently being used to scale at a significant cost and carbon impact. The cost alone places many communities in debt, but it is also unreliable when it breaks down and can take weeks to fix (I have personal experience with this). If I read your reply correctly, you agree with me that it is unlikely to deploy nuclear in locations where sufficient political or techno-regulatory requirements are not in place … which says to me it has fairly limited utility to address many of the climate and energy challenges we face today (when viewed from a global perspective). If we need affordable and scalable energy alternatives that stream well in both directions (from the Third World to the First, and back again as you suggest), and I want to highlight that “leadership” in well developed countries often has an important role to play in this, what are the alternatives as you see them?
It certainly has, and we discussed many of them above. With low cost and high emissions fossil fuel capacity ready and available on the grid, it simply hasn’t been very important up to this point in time. This is one of the main conclusions from the Science article cited by Barry (cost and not technical feasibility “is given as the reason that energy storage is not widely used on the grid”). And the cost picture is rapidly changing, along with the rules for integrating large amounts of energy storage on the grid. These costs are detailed in an EPRI white paper on EES applications, costs and benefits (from 2010). CAES (underground) is currently at $60/kWh, which is very attractive for bulk energy storage (but it is geologically limited). Sodium-sulfur batteries are being used on a commercial basis today (for load leveling, peak shaving, frequency response). I’ve presented a costing for a national deployment of current NaS above (calculated on a conservative basis). Newer experimental technology won’t be built unless it can beat out these currently available commercial options: on a cost, cycle life, energy density, discharge period, recyclability, round trip efficiency, material availability, basis. And we have many candidates in pilot or early commercial scales of development : liquid metal, flow batteries, advanced CAES, fuel cells (metal-air), hydrogen storage, and other options for distributed, commercial, residential, frequency, substation, and smaller applications. I don’t see where our choices are few or limited, but many and varied, and are rapidly being brought to scale (despite historically low R&D funding).
I agree with EL above that this thread has shown an abundance of solutions to the storage problem. But assume for a moment that the opposing view is true and there is just no way that storage can ever be deployed on sufficient scale and low cost to make wind and solar a realistic option.
Then there are essentially two ways to use this as a talking point for pro-nuclear advocacy.
The correct way to do it is shown in this recent editorial by Christine Todd Whitman.
http://k-lenz.de/1015
Point out the strong growth in intermittent sources (wind and solar). Then point out that nuclear energy is an ideal match to balance a grid with more renewable sources.
The alternative approach is to tell people to stop their support for solar and wind and support nuclear power instead. This is guaranteed to fail and will only increase opposition to nuclear. Adding the deliberately disparaging term “unreliables” will backfire even more.
There is already no lack in such opposition without such fundamental mistakes in message framing.
@EL:
Not so. I did not say and do not believe that nuclear power presents a proliferation or safety threat that renders it inappropriate for global deployment. This position is both socially intolerable and technically unsupported.
We came to this point in the discussion via energy storage. We could have arrived via a number of paths, including generation options, which is where EL has taken us in his last post, but with a nod at the end towards a shopping list of storage options which are not yet options in the wider sense for various reasons.
What I am trying to say is that I disagree with those, such as EL, who provide a constant optimistic stream of complex, currently impractical non-solutions to the world’s twin problems of climate change and energy, especially electrical energy for fixed applications and stored energy, in any form, for transport applications.
In the 1960′s, Phil Spectre became famous for devising the Wall of Sound, which helped to make many a musician famous. Characteristics of the Wall of Sound included many musicians, playing many instruments, so many that only a few can be discerned at any time. There are no gaps in the delivery – it is wall to wall noise.
The TwentyTens equivalent appears to be dominated by the Wall of Noise.
The noises from various quarters regarding climate change and energy decarbonisation have the same effect. The backing orchestra is a motley bunch, not always synchronised or even working in the studio at the same time. Like Phil Spectre did, the art of the overdub is dominant. Little bits are played and repeated, cut and pasted, so that the lead singer (main theme – climate science) is all but inaudible.
The climate backing orchestra includes:
… Those who Naomi Oreskis dubbed “obscurers of truth on issues from tobacco smoke to global warming”
… Think tanks, exposed by James Hoggan in “Climate Cover-Up” as being the as being paid participants in industry-funded campaigns to generate doubt, to astroturf their backing industries, to use courts and to spread public lies, all with the purpose to delay and to deny progress of knowledge and action regarding the imminent challenges due to anthropogenic climate change.
… Lobbyists and their disinformation campaigns aimed at preventing public and political agreement regarding responses to climate change, even where these threats have been agreed to exist.
… Industry salesfolk for specific technologies, including wind and solar photovoltaic and solar thermal and more, who, if they were selling cars, would suggest that the car they are selling has a petrol consumption figure of 10,000 kilometres per litre, because that is what they assessed it to be when coasting down a long hill, with nary a mention of the cost and effort of the other 99% of their favoured product’s performance.
To this I add:
… Those who believe that third world countries cannot be trusted with real solutions to their energy dilemma, especially those who pretend that security or political issues render impossible the provision of this or that technology. This is not only hugely insulting, but is mistaken – these issues have solutions and/or have been mightily exaggerated or misunderstood from the start.
… Helen Caldicott and her tribes of followers who imagine problems where none exist and who have for 40 years propagated untruthful messages that nuclear power is evil; that it will be the end of the world as we know it; that nuclear = weapons of terror; and that radiation, however small the dose and however delivered is intrinsically not only bad but inevitably fatal.
I now add to this wall of noise:
… spruikers for endless subsidies,
… pushers of a multitude of possible future technologies which at present are at best speculative, who thus add to the delays, the noise and the confusion. EL may be one such.
… Those who point to the partial though prohibitively expensive efforts of rich Western nations to harness wind and sun and, by extrapolation but without accounting for the limitations of these technologies and the impossibility of funding their deployment at the envisaged scale, tout them as solutions, when they are not.
… Fighters of the 1970′s arguments who build whole political platforms around notions that are frozen in the Cold War of the 1960′s and 1970′s.
… Those who envision that because the First World countries are doing something at great cost, the same pathway is available to Third World countries with almost no resources.
EL’s stream of gadgetry is not a solution and he has shown that he favours denying nuclear solutions to those who he doesn’t trust.
How, then, are those who have been denied access to their needed energy going to be kept from becoming climatic and economic refugees? What will the First World’s response to the mass migration pressures, the resource wars (Read: Gwynne Dyer “Climate Wars”), when those who have not demand fairness from those who have?
In 2009, David McKay wrote “Sustainable Energy – Without The Hot Air”, one of many available texts which address the subject of limits of development of the various forms of renewable energy. His book has been attacked, often unfairly, on the periphery, yet one core message remains and it is this – there is simply no way that renewables can, using currently available technologies, satisfy the world’s need for energy.
David McKay focusses on not only the energy needs and raw energy production potential, but also the cost, the land requirements, the possible rate of deployment, the safety, the cleanup of each of the studied technologies. He has had a pretty good go at finding the practical limits of technology, for these are very important.
Those who divert attention by wailing “Over here! Look! Tomorrow’s gadget!” are part of the wall of noise which prevents action to address the reality of climate change in every nation, right now.
My suggestion to EL is that he carefully read and digest David McKay’s book and George Monbiot’s “Heat”, both of which are massively researched and stay focussed on the art of the possible. By all means, research and design, demonstrate, develop and commercialise new options, but please realise that action today can only use the tools available today.
@ Karl-Friedrich Lenz, on 1 December 2011 at 11:24 AM:
Karl says that there is an “abundance of solutions to the storage problem”.
I do not agree that a single real world storage option has been identified here as being available and deployable at an affordable price and scale to be able to achieve either of the two main objectives, which must be:
… cheaper, reliable energy.
… CO2 emission reduction or avoidance.
Perhaps Karl could propose an practical storage option to backup ocean wind generation, or domestic storage to take houses off grid, or some other available storage option, because I simply don’t see bulk storage as a viable option for deployment in 2011.
If Karl has nothing available and ready to go, perhaps he has demonstration proposals for us to consider; proposals which are founded on costed designs with realistic potential for global distribution. Let’s suggest a timeline for progression from demonstration to full deployment at TWh rates annually, of not later than 2020, because if storage isn’t being rolled out big time by 2020, then it cannot be the foundation of today’s planning. Time is precious.
@John Bennetts
I appreciate the occasion to sum up my observations, though my point in the post above was rather how this whole storage discussion can help pro-nuclear advocacy.
First off, storage is useful for nuclear as well as renewable, since the problem is not having insufficient supply in some theoretical wind and solar only system that no one will build anyway, but what to do with insufficient demand right now. Switching off low carbon sources is wasteful.
In Germany, the solution will be making hydrogen or methane for the next couple of decades. The latter gives over 100 TWh of storage with the existing grid. As referenced above, people have started doing that already. Not because they are afraid of shortages, but because they don’t want to switch off their generators when demand is lacking.
Other solutions are electrical vehicle batteries (McKay), quicklime cycle, moving mountains, pressurized air and pumped hydro. You are of course free to assume that none of that will work. Actually, that was the premise of my last post. From your pessimistic point of view, how do you use that talking point for pro-nuclear advocacy?
@ K-F L:
1. I am a realist. I construct things that work. Proposed solutions are only dreams until the problem which they address is identified and until they can be constructed. Post-construction verification by measurement of costs and results is then possible.
2. I never said that my purpose on this thread is pro-nuclear advocacy. Let’s stick to the subject and avoid the straw man arguments.
3. Let’s try again to identify realistic answers to realistic questions. If a strong supporter of various storage options cannot provide a single real world example of where the proposed storage will be effective today, then at present there are no such options on the table. This points to the need to stop making the problem larger.
The Sahara quicklime cycle isn’t relevant, unless and until the problem which it purports to solve exists, and there is no Sahara array. Dreams.
Other suggestions such as auto batteries and compressed air have been addressed already. Their limitations have been exposed. For example, and as I said above, car manufacturers competing in the transport market may adopt differing strategies to batteries. The one with the better arrangement for transport purposes will always win market share over another which tries to saddle his car with non-transport objectives.
The example of hydrogen generation has already been demonstrated to be unsatisfactory on the basis of cost and efficiency, but it is at least a partial solution. It is not and will not soon be affordable. Perhaps, in Germany or for demonstration plants, cost does not matter. I suspect that the real cost of fiddling with dreams will become visible, even to Germans, and the hundreds of billions of Euros invested in renewables for so little gain will be subjected to harsh criticism. However, I guess that if cost is not a consideration then hydrogen generation and storage in the existing gas distribution system is up for consideration as a problem with a solution.
As for pumped hydro – that remains a wish until costed details are available. Where is all this hydro capacity waiting to be tapped? What cost? Other threads on BNC examine this. Pessimism? I’m called a pessimist because I want to know that things will work at the necessary cost, scale and time? That is not pessimism.
It is becoming clear that cost-effective storage options are not available to address (for example) the two scenarios which suggested by me – domestic PV off-grid and smoothing ocean wind generation. These are large and rapidly growing problems. Various nations are well on the way to spending many billions of dollars making these two problems worse. Both would clearly benefit if affordable storage was available.
This isn’t a discussion in a pub. There are social rules regarding claims made on BNC, including that claims made must be substantiated.
I have sought substantiation of the cost, availability, scale and so forth from two repeat posters on this thread. Neither has, with the partial exception of the hydrogen generation trials which represent a return of only a fraction on the energy invested, provided answers.
Let’s face it – today, there are very few large scale storage options with demonstrated capacity, yet some current generation strategies are increasing the problem hugely, with the result that in lieu of storage, FF backup and peaking plant is mandatory – not optional, but essential. This represents failure to achieve the primary goal, which is decarbonisation of energy.
This is not tomorrow’s problem, it is today’s. It cannot wait. Actions which make it worse must be exposed and halted. If this means that unreliables cannot, with integrity, be recommended as an adjunct to nuclear or other energy forms, then so be it.
Let’s get real – the world is facing a disaster of unprecedented magnitude, and we are discussing ways to feel good while still doing the wrong thing?
To the disaster is added a scandal, and that is that people are misled by well-meaning people about the carbon emission profiles of the unreliables industries. When will the time to face the facts arrive?
Meanwhile, I wait for K-F L to provide costs and logistics to match the rhetoric.
@John Bennetts
Your point may not be how this fits into pro-nuclear advocacy. Mine was.
If you expect me to rehash the whole discussion on individual solutions in one post, naming “costs and logistics” for each one, I am sorry to say that I won’t comply with that request. That would get quite long, repeat only what I said before, and distract from the point I was making with my post you were discussing in the first place.
(My bolding.)
The information necessary to demonstrate that storage is a panacea has not been “said before”, whether by K-F L or otherwise.
Meanwhile, the problem grows, unaddressed.
This site is not, as suggested, about nuclear advocacy. It is about climate primarily, with many threads about significant impactors on climate and a liveable world.
Nuclear power, though a common issue, is not the primary focus of BNC. Climate change is. Continuing build-out of FF gas generation is not a nuclear power problem – it is an unavoidable corollary of unreliables and of the lack of viable alternatives to gas fired plant. This is where storage becomes most significant.
As someone else pointed out, even in a nuclear + storage future, were that under active consideration, the amount of storage required would lie between 0 and 10 percent of that which would be absolutely required to replace FF backup. Wind + Storage immediately faces 10 times the storage problem. Is that not worthy of costed consideration?
That storage it is which remains to be demonstrated to be even remotely possibly, either now or in the near future.
That is why storage as an option with wind and/or solar is not currently a satisfactory proposal, whether planned for installation immediately or in the prospective future.
That, ultimately, is why I will not now accept that wind and solar are worthy of consideration as zero carbon energy sources.
That is why I mourn the waste of time and money by some large and smart nations chasing “energy solutions” which are nothing like a solution.
I’m far too honest to dress wind and solar up as the energy future of our planet. I may have ignorantly adopted this position 3 or 4 years ago, but not now. I’m far too honest to pretend that storage can get us out of the hole which we, collectively, are digging for ourselves into.
If this thread was intended to bring forth hither-to unrecognised energy storage options which can wean us off fossil fuels, it certainly has not done so thus far.
@ EL – I assume you mean CAES at $60/MWh not kWh. If the price is so attractive why haven’t more CAES systems been built? The technology has been around for over 30 years. If utilities could make money on CAES storage you would image they would build more systems.
The fossil fuel consumption of a traditional CAES facility is surprisingly large, only slightly less than a combined cycle generator (and CAES costs more than CCGT, and is more site specific). So it is quite unsurprising that it isn’t used more often.
CAES will only become globally attractive if the heat storage version pans out, at low cost.
CAES only makes sense if you have too much nuclear and gas prices are high and you have a lot of salt domes not in use. Few utilities have all three of these at the same time.
If gas prices are high, the entire CAES concept becomes uncompatitive; CAES uses luxurious amounts of natural gas (not much better than a CCGT in fact).
Salt domes can be found in lots of places, though. Deep saline aquifers are more attractive because you can compress and decompress nearly isobarically.
It doesn’t have anything to do with trust, but with the institutional supports needed to run very technical nuclear power plants on a safe and reliable basis (and also deal with all of the fuel cycle, enrichment, waste storage, energy distribution, financing and investment, and policy supports needed to pull any of it off). We have a hard enough time trying to do this in the States and attract private investment dollars to nuclear (with our international reputation for plant reliability and safety, stable banks, mature political system, insurance programs, and the like). You’re claiming I’m primarily making an anti-proliferation argument, and I am not. When most people just want to turn on some lights at night (and replace existing diesel plants), importing a whole suite of government, regulatory, debt finance (in 50 year range), bulk power systems management, waste disposal, insurance, and training programs at the post-graduate level seems a bit overkill to me (especially for a small agrarian, herding, or fishing community). This is not anywhere near a cost-effective or materially rational strategy for carbon mitigation or economic development alternatives for the Third World (at least not anytime soon). It seems to me for remote areas, or locations off-the grid (where 2 billion people currently live), weather dependent systems (with a little bit of storage) should be fine. Building industrial and GDP growth around this weather dependent infrastructure will also encourage smart growth, and very high levels of sustainable conservation, energy efficiency, and low natural resource dependencies.
Length of discharge is an important feature to understanding storage costs (which are not the same as cost of energy delivered, or LCOE). Current targets for ARPA-e grid storage projects are $100/kWh (which is seen as market penetration target for bulk energy storage applications on the grid). You’ll note in EPRI fact sheets, commercial CAES already beats that target (with adiabatic CAES currently in demo projects).
There are many market conditions that have prevented energy storage alternatives from being used on a wider scale. The ready availability of fossil fuel generation is one of them (and energy companies make large profits from energy arbitrage on this basis). The requirement of storage developers to buy energy at retail rates and sell energy at wholesale rates is another (which was addressed most recently in late October in the US with new FERC rules).
EL, explaining why he doesn’t trust third world countries to use nuclear power:
What institutional supports, etc, etc? Show me a country which has unreliable, expensive, diesel powered power in its major cities and I’ll show you a country that would be lifted enormously by a packaged, buried, set-and-forget , zero enrichment, zero waste storage 60MW NPP.
Want to get heaps of value from your development aid dollars? Spend some by providing basic energy where it is needed and earn the spinoff benefit of providing energy without carbon.
What’s not to like about that?
In order to avoid drifting right off topic, I have posted further comment on the population thread.
I’ve said it before and I’ll say it again – any decarbonisation plan that does not work in the third world won’t work.
The other post is at http://bravenewclimate.com/2011/09/19/population-no-cc-fix-p1/
CAES/pumped (underground) hydro depend upon a sufficiently large difference between overnight and daytime electricity prices. The other requirement is an assured (so reliablee) supply of overnight energy.
The former reason explains why there is no pumped hydro (AFAIK) here in the Pacific Northwest. The margin prices are beginning to diverge just enough that it may pay to upgrade existing equipment, used for almost 60 years solely for pumping, in order to create a modicum of pumped hydro; we’ll see.
These things are a lot more complicated than you seem to be aware. Let’s take one real world example, a $300 million diesel power plant proposed by USAID in Afghanistan. According to your assessment, this project should be a go (what’s that complicated about operating a heavy fuel power plant in the most prosperous and stable region of the country). Only 10% of the population currently has access to electricity, the US spent $732 million to upgrade the grid. What do we find: the power plant is now $40 million over budget, a year behind schedule, a Chinese subcontractor abandoned the project with security concerns, and the Afghan government may never be able to afford the fuel to run it, or the trained personnel to operate it on a reliable basis (given it’s “technically sophisticated fueling operation”). In short, the power plant may never run (certainly not at levels sufficient enough to meet local electricity demand), and Afghans have come to refer to the plant as “‘Karzai’s winter coat’ — designed to keep him comfortably in power [pun intentional] through the winter of 2009.”
This is not to suggest that Afghans can’t pull off sophisticated energy projects, especially when built from the ground up (and with local stakeholders fully at the table), but they are a long ways off from running a nuclear plant in a “set-and-forget” manner, and will likely burn a great deal of diesel or coal before they get to that point. Solar, with larger facilities down the line (wind + storage) may provide a much better pathway to economic development and electricity modernization in such a location, perhaps much better than ignorantly plopping down a $340 million smoke stack in the middle of a poppy farm, throwing away $700 million on transmission and distribution, and having it all rust out as an expensive monument to western arrogance and miscalculation (with wages continuing to hover around US $2.7/day for some time to come). Watch the video segment on Afghan stoves that goes with the story, there’s no shortage of creativity, resourcefulness, and innovation among the Afghan population.
There is a second use for CAES other than moving energy from off peak to on peak. Its quick start and black start generation. In a system with a large amount of wind quick start generation could be very useful for avoiding load shedding. The Germans used their CAES plant in this manner rather than to move energy. But that was long ago and I do not know their current status for use ot CAES. Someone said that gas prices had to be low to use CAES. Just the opposite can be true. A low base load generation nighttime cost when load levels are lower combined with a considerably higher natural gas price is the perfect environment to use CAES for daily peaking generation and the pumped air storage comes from the night time low cost energy. This is operationally cheaper than running gas peakers but to justify the CAES investment there needs to be a spread of the energy cost between light load and heavy load periods. I suppose wind could be used to pump up the CAES compressed air storage but I have not studied the overall economics of this usage of CAES. Definitely CAES could play a major role in making wind power more reliable. But then again its still a GHG plant which we would like to avoid investing in if possible, just for the sake of the planet, ignoring near term beneficial economics.
EL wrote
The obvious solution is to encourage the international adoption of simplified nuclear powerplants with simple passive safety features. The AP1000 is a good example, as is the ESBWR and the Kerena reactor.
Keep in mind that the issue may be entirely theoretical, since the institutional context is usually copied from the technological source country. When Canada sells a CANDU to a foreign country it gets accompanied by a big training program and hugely bureaucratic work environment. To even run a nuclear plant correctly requires a large degree of know how and operator training. If they don’t know what they’re doing the reactor or pumps or turbine will simply trip and they won’t even be able to start the reactor system.
I agree … it seems like this issue is near and dear to your heart. Here’s what a compact, mobile, and easy to install renewable energy system looks like when deployed in remote locations in Afghanistan (where it’s needed to earn trust of tribal leaders, provide local alternatives to the drug trade and insurgency, and not just further concentrate the wealth and power in urban centers like Kabul). The story of energy democratization could be told in many places (Afghanistan is not unique), and we have our own experience here in the US with depression era rural electrification, and in particular the Tennessee Valley River Authority (where renewable energy had such a big role to play). With viable and cost effective storage options, this system has even more advantages in remote locations without a grid. The video has a promotional cast, and the testimonials are a bit scripted, but the concept looks good to me, and the program appears to be offering training in skilled trades, local jobs where they are needed, and even managerial opportunities. And if you think electricity is not making a difference in Afghanistan, there’s a pretty remarkable documentary that came out a couple of years ago called Afghan Star (see trailer), which has sent people scrambling to find batteries to run their TVs for an hour during the broadcast, and is connecting people across the Country and providing a safer alternative to warfare for expressing long standing tribal and cultural differences. It’s a remarkable documentary, well worth a look for someone interested role of technology and rural economic development opportunities and living standards. I don’t know the full status of SESA (Sustainable Energy Services Afghanistan), current projects are listed here, but it would be interesting to know if they have been successful deploying these renewable energy systems, and if larger systems are planned for the future.
EL, the third world and rural electrification aren’t synonymous.
The third world is rapidly urbanizing and industrializing. Directly by having more people in cities and near major industrial areas where the jobs are, and indirectly in the sense that rural people increasingly buy stuff and goods from the industrial areas.
Rural electrification is an important human development goal. That’s not the same as saying its an important decarbonization goal. The amount of energy used rurally will always be minor. Where it is bigger it is from centralized fossil sources, eg nitrate fertilizer, diesel powered machinery, etc that is being used to cultivate. There’s a huge centralized fossil infrastructure behind all of this. Even rural areas have many connections to the urban, industrial spheres.
Human development, in this case using whatever technologies that work to electrifiy the countryside, is important. At the same time we should not confuse the task of decarbonizing our current and developing future predicament. The idea that including rural electrification will solve the decarbonization issue is simply wrong and misleading. We will need almost all energy provided on an industrial scale, because almost all fossil fuel is being used on such a scale. Replacing diesel generators with solar and wind-battery hybrids for remote powering is great, but don’t kid yourself that most diesel is used for this. It isn’t.
@EL
Thank you for that interesting link to the Afghanistan project. I enjoy reading your contributions and often find much to learn in the links you provide.
I thought that I had made clear that I consider large scale power generation only viable for cities, yet EL has again produced a straw man argument.
NOT rural, especially when the bulk of folk go to bed with the sun and industrial load is minimal. That was a straw man argument from EL.
YES efficient reliable power for larger towns, cities and industries.
My point was and is that complete energy solutions for 24/7/365 loads must come at a price that Third World countries can achieve, ie the option with the lowest overall cost. No solution to the world’s climate problems will be found while western nations remain focussed on expensive add-ons while the majority of the world’s population remain locked into carbon-based energy.
This thread is not about energy options for poor rural communities. That discussion is better suited to the population thread.
Not meant like an ad hominem, but I for one do not enjoy EL’s contributions. He or she is constantly pulling up smoke screens with some kind of reference information overload tactic (witnessed by the fact that EL often doesn’t know what is being said in the references). EL is trying to win some battles, not realizing he or she is losing the war.
Here’s one of the main reasons we’re losing the war:
http://gregor.us/wp-content/uploads/2010/02/Developing-World-Coal-Use-1989-20081.jpg
And a projection based on that simple trend:
http://photos.mongabay.com/09/coal_1990-2030.jpg
Developing world coal use is skyrocketing. This coal is not used in small romantic villages to cook some beef. The developing world is rapidly developing, that’s why it is called developing world. Their needs are increasingly like the need of more industrialized countries. Their trajectory is similar to the industrialization of Europe and the USA, albeit with more modern means to use the fossil fuels than James Watt’s steam engine.
Without cheap scaleable energy storage, renewables can’t do much against coal use rising. That’s a simple fact. And even with cheap storage, what’s to stop developing countries from using cheaper, reliable coal power rather than expensive, unreliable solar and wind?
The clearest solution to this problem is to provide energy cheaper and more reliable than coal. Nuclear fits the bill, but only if we are serious about it, like France, unlike the USA which is bent on red-taping to death any new nuclear initiative.
MODERATOR
Each to his own Cyril – but it gives you an idea as to why it is difficult to moderate some comments.
Fair enough moderator, I’ll try to focus on the ball some more.
I posted this comment in another thread but it is better suited here.
To get enough power for all existing electricity supply plus electric vehicle charging plus heat pumps to heat your home and provide hot water, requires an average flow of 1.5 kW of electricity. One week of that is 252 kWh of electricity storage. Realizing the heat pumps and electric vehicles could be used for effective load deferring, we can remove about one day of energy storage, leaving 6 days. That’s 216 kWh that we need. If it is 90% efficient that we need an extra 10%, 216/0.9 = 240 kWh.
The best NaS cells get 240 Wh/kg (coincidence that this is the same figure, funny world isn’t it?). 240/0.24 = one metric ton of batteries per person. This one ton battery per person needs to be replaced every 10-14 years at least (cell float shelf life).
http://www.google.nl/url?sa=t&rct=j&q=nas%20battery%20kwh%20kg&source=web&cd=6&ved=0CFYQFjAF&url=http%3A%2F%2Fwww.iea-pvps.org%2Findex.php%3Fid%3D3%26eID%3Ddam_frontend_push%26docID%3D744&ei=DgDZTp7DCoGYOpqj5K8O&usg=AFQjCNFwUTeRrB7Fjnm7BtidlvkDaeVADw&cad=rja
The cost of this battery, at today’s $300/kWh, is $72000 per person. At an optimistic future cost of $100/kWh, it is still $24000 per person. This is a recurring cost, every 10-14 years you need to buy another $24000 battery.
—————————————-
I wanted to find out how much energy would be required to make this battery. One ton of battery cells per capita is rather much, and making sodium and sulphur, and structural holding materials plus ceramics/membrames etc, is energy intensive.
Cyril, that roughs out to $2k to $6k disposable income per person, per annum, just for energy storage. It can’t be done.
Add the necessary PV panels or whatever, and it is clear that battery storage isn’t a general solution – it is only likely to be fit for niche uses – eg transport, and then only where daily off peak recharging is both reliable and economical.
Given that most electricity is not supplied for domestic use but for commerce and industry, why has much of the focus been on domestic rural solutions? This avoids the real problem. Why bang on about sustainable off-grid partial solutions, when the grid is essential and will remain essential for decades or for ever? Is it because individuals are more susceptible to advertising hype and emotion in their decision-making than are organisations which understand the costs and the negative flow-on implications?
Somebody up-thread stated that placing a smokestack in a poppy field could not work. As if covering many more poppy fields with mirrors or PV panels would be any more welcome! This was a classic straw man argument.
That this thread has become a bit of an emotional slanging match is unfortunate, because the energy and climate solutions which are fundable today cannot be founded on untested or experimental or hypothetical technologies. Yet, today is when the solutions are needed. Tomorrow will have its own challenges and there is no doubt that some of these notional technologies will eventually bear fruit.
To be achievable, these energy and climate options, including storage options, must, as Cyril mentioned above, work for developing countries.
It is abundantly clear that loopy “just do it” type of thinking is extremely wasteful. Consider, for example, the huge expense on wind and PV, which has necessitated backup gas turbines, then transmission line upgrades and now we are considering storage options. None of these consequential actions was part of the original decision to plonk wind turbines across the landscape, yet they are now essential. They should all have been included in the cost of the very first wind and solar roll-outs, but the “just do it” brigade in Germany, Spain, USA and, indeed, almost every nation world-wide, whether “developing” or not, succeeded in obtaining emotional rather than rational decisions. This band of happy sales folk are unrelenting in their determination to privatise the profits while foisting the costs of onto the public and to governments.
After 190 comments, nobody has brought us a deployable storage solution which is cheaper than a lead acid battery.
It is incumbent on the proponents of emotional energy sources to include storage in their costings. If conventional baseload (nuclear and coal) need only 10% as much storage, then the cost of the additional storage is part of the cost of the unreliables. This thread has demonstrated that storage costs are another nail in wind and solar’s coffin.
Developing nations cannot afford failure on the scale of a Desertec.
On 13th November, Max described an electricity-to-methane trial in Germany with 35% efficiency. That isn’t the end of the story, because by the time that this is converted back to electricity via CCGT the round trip efficiency is close to 10%. For this to be worthwhile, surplus variable electricity would have to be extremely cheap – essentially, given away. I’m sure that the owners of the solar panels and wind turbines would hope for a better reward. However, Max’s example serves to demonstrate that much of the energy produced by variable sources is absolutely worthless unless and until technological advances result in 90% efficient storage, in bulk, at low cost. The response from purveyors of renewables has been to obtain legislation in many jurisdictions which forces grid managers to place wind and solar first in the queue, regardless of price and consequent cost. That serves only the industries which supply renewables and is a scandal. They are freed to pretend that the storage problem isn’t theirs, but that it belongs to the grid managers and, ultimately, to all electricity consumers. This is insane.
Sadly, this thread has shown that practical storage options are out of reach.
Practical solutions must be found elsewhere, starting with addressing the root causes of the need for more storage. We have the concept of Negawatts. How about Negastorage, which is one benefit from disconnecting unpredictable electricity sources from the system?
Cyril, did you read my reply in the other thread? Do you have a reply to my comments? (Deleted inflammatory remark). The costs as you describe them have nothing to do with credible research in this area, modern electrical grid operation, capacity planning, or energy storage applications (as they have been described extensively in several sources listed above … DOE, EPRI, Sandia, NREL, professional workshops, or elsewhere. Here is what I wrote to you in the previous thread:
I also provided a costing for a storage system using current technology and costs that would be sufficient for a high renewable option for the entire US electrical grid (45% from wind and solar, 10% from hydro) drawing on conservative assumptions.
Please redo your analysis using conventional approaches to modern power systems management, grid operability, capacity reserve planning, current technology availability and costings, and plausible pathways for energy storage scalability and commercialization.
The issue of rethinking energy delivery with storage in the mix is indeed a challenge, since most of our energy markets are designed with load following and flexible fossil fuel reserves as a basic element or backbone (see recent FERC ruling to looks to change this). What do you consider to be “out of reach costs?” Is $10 per person/year impractical (as I have suggested above). And this is for a maximum approach to energy storage. The cost for a minimum approach (of 10GW storage over 10 years in US, recommended in DOE resource planning estimates) would be around USD $3.30 – $4.90 per person/year (based on hybrid model of storage proposed above). Impractical estimates follow from impractical assumptions. I’d be interested to hear your view.
This is not correct. The American Society of Civil Engineers gives the transmission grid in the US a D+ rating in it’s Energy Infrastructure report card. These upgrades are long overdue, and would be required regardless of sustainable resources being added to the grid. They point to growth in electricity demand, congested paths or bottlenecks, low annual investment in T&D, “incomplete transition to fair and efficient competitive wholesale electricity markets,” and not renewables energy resources as basis for their low grade. In fact, they specifically identify “solar, wind, hydro, biomass, and geothermal” as part of the solution (and not the problem).
EL wrote
The “conventional approach” is all about using lots of fossil fuel. This is exactly what we SHOULD’T be doing.
You think 45% renewable is clean. I think the other 55% is almost exclusively fossil fuels and that is not acceptable in a world with rapidly growing energy demand and emissions.
In a renewables grid, minimum energy storage = maximum fossil fuel use.
There are no plausible pathways for energy storage scalability at the scale that is required to largely eliminate fossil fuels in electricity grids. That is an important take-away message from this thread, which you have utterly failed to grasp.
This is not a plausible benchmark (as you have identified it). If the “perfect is the enemy of the good,” none of our energy sources qualify, since even nuclear in very high levels of penetration emits a minimum of 60 – 65 gCO2e/kWh, and also requires integration and balancing support from flexible resources (as the French case illustrates with 15% from coal and natural gas). And let us not leave out that this technology pathway has significant hurdles to clear with respect to private sector involvement, financing, low public acceptance, fuel cycle development, waste disposal, national security, regulatory pre-requisites, scalability to non OCED countries, and more. What are your assumptions, you have not identified a plausible carbon reduction goal in your statement. If the goal is to reduce carbon emissions by 80% below 1990 levels, then doing so with a large share of renewables and enabling technologies such as energy storage does appear to be feasible, and is recommended by some as a viable pathway (with respect to costs) for deep GHG reductions. These issues are challenging, defining them such that no goals can be reached is not a useful approach to the problem.
MODERATOR
EL – Cyril R is right- this thread is about
You are veering off topic on this thread when you introduce perceived hurdles to nuclear power and other red herrings. Please continue that line on the Open Thread
EL: it’s useful to have the range on ghg emissions for current reactors, and note that generation four would close the fuel cycle.
Low public acceptance is no reason to reject nuclear power if that low public acceptance is based on falsehoods; if “renewables” ever approach scale, there will be low public acceptance, and for good reasons.
From the sydney report you cite:
Similarly,
– accepting the qualifications and omissions stated,
– for grades of average ore bodies mined today, and
– for state-of-the-art reactors and uranium processing facilities,
the greenhouse gas intensity of nuclear power is
– around 60 g CO2-e/kWhel for light water reactors, and around 65 g CO2-e/kWhel
for heavy water reactors,
– slightly higher than most figures reported in the literature, because of omissions in
the nuclear fuel cycle and upstream supply-chain contributions,
– varies within the range of 10-130 g CO2-e/kWhel for light water reactors, and
within 10-120 g CO2-e/kWhel for heavy water reactors,
– lower than that of any fossil-fuelled power technology.
since upthread, you appear not to oppose nuclear, why don’t you support it in conjunction with a renewables build? at least the nuclear would firm the renewables and not fossil fuels.
I would be interested to know what efficiency costs there would be in running nuclear at 50% and “renewables” at 50% (compared to different mixes, greater concentrations of nuclear).
MODERATOR
See my advice above to EL.
EL is complaining about 15% fossil in the French grid, but thinks 55% fossil in his total fantasy 45% wind and solar proposal (not done anywhere in the world, nowhere close) is fine.
Then he changes the subject from energy storage requirements of a fantasy wind and solar grid that is actually 55% fossil powered, to GhG emissions (on which he has no argument whatsoever, since neither nuclear nor wind nor solar are large CO2 emitters). Its not the nuclear or solar or wind CO2 emissions you have to worry about. Its the massive fossil grid behind your pretty solar panels and wind turbines that you have to worry about. Which is much more substantial for wind and solar than it is for nuclear.
MODERATOR
I have advised EL he is off topic and required him to move further comments of this nature to the OT.
The problem I have with EL’s analysis of storage cost is his use of capital cost per unit of energy storage capacity ($/kWh). This measure tells us nothing about the cost per unit of energy delivered from the storage which is the cost used by utilities when deciding if storage is a financially attractive option.
In my table posted on 18 November I used a cost per charge/recharge cycle in $/MWh delivered. For example, in the case of NaS storage EL’s cost is $300/kWh capacity but my cost is $80-250/MWh delivered by the storage.
For utilities, storage is just a way of delivering electricity supply – just like a gas peaking plant is a way of delivering supply. Current gas costs might be between $60-90/MWh delivered. Storage will only be built once storage delivery costs can compete with generator alternatives.
On paper both pumped-hydro and CAES look attractive on a cost basis but they are restricted by the suitability of sites. Lead acid batteries cost between $200-1,000/MWh delivered so are too expensive today for stationary storage. NaS at the low end might be attractive in some cases – particularly if a significant carbon price is introduced.
Perhaps EL would like to recalculate his storage cost based on stored energy delivery costs rather than capacity costs and see if it is still attractive.
Gregory Meyerson — I”m not sure I understand the question: I would be interested to know what efficiency costs there would be in running nuclear at 50% and “renewables” at 50% (compared to different mixes, greater concentrations of nuclear). Nonetheless, an attempt, which also partially addresses storage costs using conventional pumped hydro.
Following the Doty Energy figures for new pumped hydro from a link in a prior comment, the LCOE of delivered energy (US$/kWh) is
LCOE(out) = 0.055 + COE(in)/0.8
so with a firm contract to buy overnight (8 hours) from NPPs @ 0.05, the pumped hydro facility has to sell during the daytime for 0.1175 [busbar]; that’s expensive. During the daytime (16 hours), the NPPs have to sell @ 0.089 to meet the required LCOE average of 0.076 (VC Summer LCOE).
Assume solar PV can handle the entire daytime excess load (above that supplied by the NPPs) for 4 hours every day [with minimal backing from natgas]. That leaves 12 hours to be met by the pumped hydro.
Wind is too variable to bother with.
Without having an reference grid I can’t do variations on this theme, but this will suggest how the considerations have to go.
@EL:
Compare and contrast the following two quotes.
By departing from the words used, EL has intentionally placed an entirely different meaning to that which was conveyed in ASCE’s original. This is not only disappointing, it is intellectually dishonest.
ASCE were discussing an amalgum of well over 3,000 poorly-connected separate grids in USA, a situation which has been recognised for decades as being unable to deliver the efficiencies and reliability which better integration could provide, using only the existing generating plant. This arrangement is also anti-competetive in many locations. I suggest that the reserve margins referred to in the first part of the correct quote are achieved by using spinning and standby reserves which exist in one part of the country to meet unmet demand in other parts of the country which are not effectively interconnected. ASCE recommends that these interconnectors should be constructed.
ASCE then moves on to consideration of new generation opportunities. Interestingly, where ASCE lists “…highly efficient fossil and nuclear and renewable technologies…”, EL has omitted mention of fossil and nuclear from his list of highly efficient generation options. This outrageously selective and intentional misquotation is, unfortunately, too often evident in contributions on other sites which place opinion above truth. On BNC, the comments policy should prevent misrepresentation of the cited material, but the Moderator of has limited time to spend checking references – it is up to the contributor to keep things fair.
EL then appended his personal opinion that renewables are “not part of the problem”. This is not supported by ASCE’s article and should have been properly identified as being personal opinion or simply conjecture – or omitted entirely since it is unsubstantiated nonsense.
John Bennetts, a most telling critique of EL’s comment. I will be interested in the response, if any. Quotations taken out of context and personal opinions being offered as authoritative statements are not looked upon favourably on BNC, as you note.
MODERATOR
Thank you JB for supplying this information on EL’s comment. Do you have the date and time of the comment to which you refer? I can’t seem to locate the original.
Time constraints make it impossible to check out each and every link/ref (other than they exist) and claim by commenters. EL knows he must abide by the Comments Policy and he is now on permanent moderation. I ask any commenter who discerns similar violations in any post to notify BNC immediately for moderation.
I beg to differ John. I have read the ASCE’s article, and everything I have stated is FULLY SUPPORTED in the article. No inaccuracies and no misrepresentations. I described fully their basis for giving such a low rating (which you summarize in the same terms above). And I state that they view renewable generation as a part of the solution, which they do. Where I have erred in these statements!! You fault me for leaving out that nuclear and efficient fossil generation is also seen as a part of the solution. Well, that is not a question I was looking to answer, I was proving a specific rebuttal to your claim about renewables and that transmission upgrades were not seen as “essential” prior to the “original decision to plonk wind turbines across the landscape.” The ASCE article clearly shows that line upgrades were viewed as essential prior to building wind turbines (which you seem to agree with me on), and that renewables are viewed as a part of the solution. These are factual statements, and they accurately represent the statements in the report. The article also mentions a number of other solutions that I did not mention: better maintenance of existing infrastructure, operation and control schemes, transmission support for adequate reserve margins, energy conservation, additional R&D, and public education. I did not mention any of these solutions either, because they were not a part of the question I was answering, which was specifically a response to your statement and the role of renewables as described in the ASCE assessment.
To the moderator: please take me off permanent moderation (since there is no basis for doing so). It would be an odd precedent to place someone on permanent moderation simply because they have made an accurate statement, and provided a specific response to an issue raised by another member. John Bennetts appears to agree with me on my characterization of the ASCE report (even though he might not know it himself based on his reply to my comments above, and the tone that can be inferred from those comments).
MODERATOR
It is Barry’s decision whether someone remains on moderation. You are not the only one whose comments are held in the Pending file for review, for a variety of reasons.
David B: thanks for your reply. I get your numbers.
I’m wondering what a “compromise” between nuclear and renewables (wind and solar) would actually look like if both provided 50 % of a country’s electricity. Could you even do this with just nuclear and overbuilt renewables, or would you need gobs of storage anyway, charged by both the excess electricity from renewables and night nuclear?
same problems would occur with gas at high penetration. how inefficient would the gas have to be if it had to firm very large amounts of wind/solar? (50% of actual electricity in a country with large electricity output, 250 Gwe average).
I guess this is Peter Lang territory. what difference would it make if nuclear did what gas does in Peter’s models?
Hope this way of putting the question is coherent.
@gregory meyerson
You might find “ANALYSING TECHNICAL CONSTRAINTS ON RENEWABLE GENERATION TO 2050″ prepared for the UK Climate Change Committee by the consultancy POYRY to be useful. It can be downloaded here http://www.theccc.org.uk/reports/renewable-energy-review
Unfortunately the report is based on proprietary modelling software, so it is a bit opaque.
All the documents related to Renewable Energy Review are well worth reading. It seems they assume good flexibility and load following capability in new nuclear in the UK.
EL:
Selective quoting does not fairly represent the original author’s opinion as presented in the referenced article.
To ensure that readers such as I are not mislead/confused when citations are longer than just a phrase or two, it is good practice to use the blockquote formatting function to display the citation without alteration or omission, within inverted commas. This you did not do.
Any omissions from the cited text should be indicated by insertion of ellipsis (…), taking care to ensure that by so doing, the original meaning is not distorted. Omission of the first few items from a list is rarely fair, for by common usage, the first items are often those which are afforded the most weight. (For example, by omitting fossil and nuclear fuels from a list which then includes a range of renewables the weighting allocated to the remaining fuels is not that of the original. It is not a fair citation.)
In situations where any doubt could arise, any personal interpretation should appear in the subsequent or preceding paragraphs. Use of the blockquote function assists greatly to separate the citation from the comment.
These techniques can lead to improvements in:
… Factual rigour
… Logical organisation and presention of thoughts; and
… Readability.
Most universities and faculties have quotations guides for use by authors of theses and reports. If you wish, I and others will provide links to suitable guides.
I will make no further direct comment on this issue.
JB
gregory meyerson — Everybody seems to be of the opinion that 50% [nameplate] penitration by wind+solar would cause an unreliable grid, highly prone to blackouts. This can be resolved with enough pumped hydro as Denmark backed by Norway illustrates. That unique situation probably cannot be duplicated anywhere else in the world.
With current prices for the various technologies, one can hardly better the French solution [which encorporates a modest amount of pumped hydro.]
A more complete survey belongs on the open thread as it goes far from the topic of this energy storage thread.
NO original meaning was distorted by my statement. The burden of proof rests with you, John (and you have pointed out nothing that is factually inaccurate in my statement). If I had made a claim that nuclear or energy efficient fossil fuels would not contribute to transmission improvements (and in particular to relieving transmission congestion or bottlenecks), you would have a point. But I made no such claim. The moderator has requested that the original comment be located. Here it is:
There is nothing inaccurate or misleading in my reply. It is specifically addressed to your concern regarding renewables and your claim that line upgrades are now “essential” as a consequence. I gave a full and accurate description of why ASCE gave the US grid a D+ rating (and renewables were not cited as a concern, but a “solution” in their assessment). They likely did this because they were reporting on transmission adequacy, and not generation alternatives (and the fact that distributing generation probably DOES improve transmission congestion). They also stated these problems have been long-standing (and we now have agreement on this). So what exactly is your objection (that I did not include extraneous information that you wanted included in my comments)? This is not an honest, or an objective appraisal, John … and I have a pretty good sense you know it. It’s my hope that the moderator can see this as well, cut to the chase (without wasting anybody else’s time), and remove any punitive measures when none have been warranted in this case.
EL, JB, let’s close this matter now and move on. Thanks.
Thanks Quokka. will study the link. david: do you have any sources you especially value, beyond the work done on this site (which I already know)?
All I can do is speak for the renewables portion of your question (and where storage becomes very important). There is an abundance of research on the costs of integrating wind (balancing and efficiency losses) for penetrations in 20 – 30% range (here or here). Much above this is hard to find, because storage becomes so much a factor. As described by Hoogwijk 2006, “Beyond 30% of present electricity production, cost increases most significantly because of discarded wind electricity, excluding storage” (p. 1382). The Irish grid is looking to become a test case for some of these high renewable energy portfolio approaches (in a fairly isolated grid context). The government has currently set a 40% renewables target by 2020. Mark O’Malley (at University College Dublin) is doing some very good work trying to model these proposals for integration (efficiency) and reserve capacity costs. I point you to his detailed on-line powerpoint, and his peer reviewed study in IEEE looks very closely at Portfolio 5 (wind to 34.3% and total renewables 42%). Tuohy and O’Malley 2011 add to this with a look at storage and integration costs for a 48-51% target, and I have discussed this paper extensively here. IEA Wind is specifically looking at high wind penetrations and integration costs (from a integration and grid reinforcements perspective), and all of their papers are available on-line for review. These are very detailed studies, and would take some time to summarize fairly and accurately. For the 10-25% range, I am confident stating that costs attributed to efficiency losses are in the 1–4 €/MWh range (roughly 10% the wholesale value of wind) as reflected in the studies mentioned above, and would require little in the sense of storage or new capacity additions.
gregory meyerson — I try to post everything of interest regarding electric grids here on Brave New Climate.
EL: thanks. have a lot of reading to do.
Sure, if you have 75-90% fossil fuels to burn, you can easily make the “wind grid” work. Of course it wouldn’t be a wind grid, it would be a fossil grid with some wind turbines for greenwashing.