In the previous TCASE post, I considered how various low-carbon energy technologies meet the following criteria: commercial readiness, scalability, dispatchability, fuel constraints, load access, storage requirements, capacity factor and emissions intensity. Here I consider the next issue: cost of deployment, based on expert consensus.
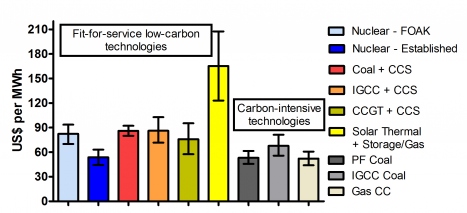
Emission intensity for fit-for-service baseload electricity generating technologies. Error bars represent 90% confidence intervals for the mean (bar height). NOTE: PF Coal = Pulverised fuel black coal, CCGT = Combined cycle gas turbine, IGCC = Integrated gasification combined cycle, CCS = carbon capture and storage, FOAK = first of a kind, CC = combined cycle.
The primary data again come from the work I had published in 2011 in the peer-reviewed journal Energy (with colleagues Martin Nicholson [lead author] and Tom Biegler). Cost was analysed on the basis of 15 comprehensive levelised cost of electricity studies published over the past decade. The data are as follows (see also figure above), with references given in the footnote:
(LCOE = levelised cost of electricity (in 2009 US$/MWh) — see footnotes for a more detailed explanation.)
Enthusiastic supporters of various renewable energy technologies have long made claims that all or most of the world’s electricity needs could be met with renewable energy. Our analysis point to the costs involved and hence to the reliance on future major advances on that front in order to be competitive with other, low-emission, alternatives. In our view such reliance is highly speculative and risky as part of any plan to secure future energy.
The hard reality is that there are reasons why diffuse and variable energy sources like solar and wind may never compete on costs. They require large installations covering extensive land areas, with costs mainly in the realm of civil engineering works and therefore not amenable to substantial reduction through advances in the energy conversion technologies themselves. Drawing analogies with ‘Moore’s Law’ (rapid decline in the cost, and increase in the performance, of computer chips), as is sometimes done with renewable technologies, is therefore not appropriate. These technologies are undeniably elegant and innovative; their limitations unfortunately arise from the inherently diffuse and intermittent nature of their energy sources.
One technical study we covered assessed wind with storage against IGCC with CCS. The wind/storage (compressed air) solution could only compete at a carbon price above $350 a tonne of carbon dioxide, well above anything being contemplated. Enhanced Geothermal Systems (hot dry rock fracturing) is a possible future baseload technology, but it is still too early to estimate performance and costs with the degree of reliability we required.
The levelised cost of electricity, shown in the figure and table above, is a good indicator of the average wholesale price the power station owner would need to break even. If an emissions price (e.g., carbon tax or emissions trading system) were introduced, the cheapest non-nuclear solution would first be combined cycle gas turbine (natural gas) with carbon capture and storage. To justify building either of the coal technologies (PF or IGCC) with carbon capture and storage for new plants would require a carbon price over $40 (or a relative rise in the gas price). Retrofitting existing coal plants with carbon capture and storage might have different costs.
If nuclear energy were adopted for the first time by a country (or if new nuclear builds were initiated after a long period of construction quiescence, like is the situation in the U.S.), its initial cost (termed ‘first-of-a-kind’) would be about $30 per MWh higher than what it would eventually reach as more plants were built (settled down costs).
The problem is, carbon capture and storage may only make sense if you take a short-term view of emission reductions. While it might deliver the probable reduction targets until 2030, if the technology proves feasible at large scales (still undetermined), the current methods used for CCS will not deliver the tougher emission targets recommended by the Intergovernmental Panel on Climate Change (IPCC) for 2050 and beyond. Coal plants often have a 40-year life, so new coal plants with CCS built over the next few decades may still be operating by 2050, holding us back from meeting those greenhouse gas mitigation targets, unless they can be modified later.
The only renewable technology that meets the fit-for-service criteria explained in TCASE 13 was solar thermal with heat storage and gas backup for cloudy days. According to the meta-analysis data, using solar thermal power to replace coal would require a carbon price greater than $150. The solar industry is ever hopeful that costs will fall, but current costs are about twice other low-carbon alternatives so they have a long way to go. Future cost reductions for any technology are inherently uncertain and should not be relied on.
The standout technology, from a cost perspective, is nuclear power. From the eight nuclear cost studies we reviewed (all published in the past decade, and adjusted to 2009 dollars. see footnotes), the median cost of electricity from current technology nuclear plants was just above new coal plants with no carbon price. Having the lowest carbon emissions of all the fit-for-service technologies, nuclear remains the cheapest solution at any carbon price. Most importantly, it is the only fit-for-service baseload technology that can deliver the IPCC’s 2050 emission reduction targets.
The relatively low cost for nuclear electricity may surprise some. Nuclear plants are often attacked for being very expensive to build (high capital costs and significant finance burden). But electricity costs are a function of construction costs, running costs (operations, maintenance and fuel) and the total energy generated over the plant’s lifetime. Nuclear fuel costs are relatively low compared to coal or gas (very little fuel is used in a nuclear plant) and these plants typically have a long life and high availability. These factors lead to a low electricity cost over the nuclear plant’s lifetime. They also offer some protection against the uncertainty of rising fuel prices for coal and gas.
The results of this survey represent the scientific/engineering/economic consensus of the world-wide, authoritative, peer-reviewed energy literature. Given the importance of reducing electricity generator emission, and the economic imperative to keep electricity costs at a minimum, it seems essential that the governments look closely at their nuclear power strategy, as some parts of the world (e.g., China, India, South Korea, Russia) are already doing.
The above assessment focuses on costs of replacement for coal and gas generation in fit-for-service baseload electricity plants. For an assessment of the cost of other technologies, including those which do NOT meet the FFS criteria explained in TCASE 13, see the following 2016 projection done by the US Energy Information Administration:
——————————-
Footnote – explanation and references for LCOE table and figure:
Note on LCOE: This is a widely adopted metric for comparing the costs of different power generation technologies. Typically the levelised cost methodology discounts the time series of expenditures to their present values in a specified base year by applying a discount rate and then divides the total discounted expenditures by the total energy production adjusted for its economic time value. LCOE reflects the constant real wholesale price of electricity that recoups for the investors the overnight capital costs of constructing the plant plus operating, maintenance and fuel costs, taxes, interest and other borrowing expenses. The cost is for the net power supplied to the station busbar where electricity is fed to the grid and does not include transmission costs or utility profit margins.
Discount rates, overnight construction costs, lifetime of the plant, energy generated and fuel costs vary across the literature depending on region of origin and pricing year. These variations account for some of the differences in LCOE values for ostensibly the same technology. For example, technologies that have relatively high construction cost, long lead time and long expected lifetime, such as nuclear power, are particularly sensitive to discount rates.
Literature assessed in the energy costs meta-analysis
ANSTO. Introducing nuclear power to Australia, http://www.ansto.gov.au/__data/assets/pdf_file/0016/12445/nuclear_options_paper_Gittus_complete.pdf; 2006.
IPCC. Carbon capture and storage, http://www.ipcc.ch/pdf/special-reports/srccs/srccs_wholereport.pdf; 2006.
MIT. The future of nuclear power, http://web.mit.edu/nuclearpower/pdf/nuclearpower-full.pdf; 2003.
MIT. The future of coal e options for a carbon constrained world, http://www.ipcc.ch/pdf/special-reports/srccs/srccs_wholereport.pdf; 2007.
MIT. Update of the MIT 2003 future of nuclear power, http://web.mit.edu/nuclearpower/pdf/nuclearpower-update2009.pdf; 2009.
NEEDS. Final report on technical data, costs, and life cycle inventories of solar thermal power plants, http://www.needs-project.org/docs/results/RS1a/RS1a%20D12.2%20Final%20report%20concentrating%20solar%20thermal%20power%20plants.pdf; 2008.
NREL. Assessment of parabolic trough and power tower solar technology cost and performance forecasts, http://www.nrel.gov/docs/fy04osti/34440.pdf; 2003.
NREL. Biomass power and conventional fossil systems with and without CO2 sequestration, http://www.nrel.gov/docs/fy04osti/32575.pdf; 2004.
OECD. Projected costs of generating electricity. 2010. https://www.iea.org/publications/free_new_Desc.asp?PUBS_ID=2207.
Royal Academy of Engineers. The cost of generating electricity, http://www.raeng.org.uk/news/publications/list/reports/Cost_Generation_Commentary.pdf; 2004.
San Diego Regional Renewable Energy Group. Potential for renewable energy in the San Diego region. Appendix E; 2005.
Succar Samir, Greenblatt Jeffery B, Williams Robert H. Comparing coal IGCC with CCS and wind-CAES baseload power options, http://www.princeton.edu/~ssuccar/recent/Succar_NETLPaper_May06.pdf; 2006.
Tarjanne R, Luostarinen K. Competitiveness comparison of the electricity production alternatives. Lappeenranta University of Technology; 2003.
University of Chicago. The economic future of nuclear power, http://www.ne.doe.gov/np2010/reports/NuclIndustryStudy-Summary.pdf; 2004.
US Energy Information Administration. Annual energy outlook, http://www.eia.doe.gov/oiaf/aeo/electricity_generation.html; 2010.
Filed under: Emissions, Nuclear, Policy, Renewables, TCASE
It should be pointed out that the levelized cost of renewables, is only the beginning of the story. The electrical consumer gets other expenses in a renewable based electrical system including the cost of Renewable generator redundancy, the added cost of electrical transmission, and the cost of energy storage. In addition many renewable advocates acknowledge a continued need for fossil fuel generation capacity. The indirect cost associated with CO2 emissions from burning fossil fuels to produce electricity has to be included in any overall assessment of Renewables cost.
Q1. Why are you using the Dec 2009 DoE Outlook 2010 when the Dec 2010 Outlook 2011 has been out for 8+ months? (Wind/geothermal cheaper than Nuclear, Solar PV halved in price).
Q2. If HDR is not ready then why is that not the case of CCS?
Q3. A good wind site is a good wind site for a number of equipment lifetimes. What allowance should be made for reuse of site and infrastructure plus distribution corridors? Same for CSP – foundations in hot-dry areas are likely to be re-useable for many iterations of plant.
Q4. Using the DoE nuclear capacity factor of 90% (too low) there must therefore be backup, or excess capacity to cover, do you agree?
Q5. What value to do you put on efficiency? ie The value of energy not needed, or capital/fuel not spent to provide new energy?
What is the point of this exercise? Stop all deployment of renewable energy and invest everything in nuclear?
That won’t happen. So opposing renewable energy will only benefit fossil fuel interests, just as opposing nuclear does.
As far as I am concerned, any price for carbon free energy is a great deal compared to the various problems associated with global meltdown. I heard these might turn out to be rather severe.
Masayoshi Son wants to invest 8 trillion yen in renewable energy in Japan. He knows that it is still expensive, but he says that you can’t choose your way to drive by looking in the back mirror. I agree. Prices for renewable have come down massively and will only continue to do so with more mass production.
Karl, there’s no reason to believe that telling the truth about renewable energy will result in more fossil fuel use than not telling it, as renewable energy is used as an excuse to not build nuclear plants.
That has to stop. What we want is for people to run the numbers, all the important numbers and not some conjured up half truths, so that they will see what we see, which is that renewable energy is incapable of replacing fossil fuels and we need nuclear.
The future is not carved in stone. It won’t happen, oh sure, we won’t replace coal, we are all doomed, let’s stop posting here then huh? Let’s go to a tropical island and drink pina coladas since none of our effort can lead to a better future.
If you worry about global meltdown, solar and wind without nuclear is what will cause it. Unreliable power that is not there 80% of the time and cannot be controlled is a good way to lock yourself in fossil fuels indefinatively.
In a world of limited resources, which happens to be the case for our planet just like any other, we have little time and effort for marginal technologies.
Germany has spent a 100 billion in solar PV and has not reduced its fossil fuel usage, in stead it has locked itself into dangerous fossil fuel backup to cover the fact that their precious solar panels are not there, on average, 90% of the time. Japan is about to do the same and you consider this a good thing? Squander several more decades in marginal technologies and use that as an excuse for no new nuclear builds?
Highly recommended and much needed perspective by NNadir:
http://www.dailykos.com/story/2011/07/24/987836/-Were-the-Japanese-Engineers-Who-Built-Fukushima-Incompetent?via=blog_658234
The LCOE figures look pretty good to me. However there is danger in putting too much emphasis on building only generation having the lowest LCOE. That is happening here in Texas (ERCOT). The only generators that are being constructed that have a chance in our energy only market are CC gas plants. They come in as mid stream dispatch generation and can effectively compete with both base load and peaking generation. This means that a new cc gas plant comes into the mix and pushes a peaker out the top, making it no longer economic, so that least efficient peaker is retired or mothballed. The economists like this. The engineers see that this results in a lack of reserve capacity so that during peak load periods, there is not enough generation capacity. Furthermore the CC gas plant drives away new base load capacity. Therefore, the CC gas plants are eroding the diversity of fuels (coal, nuclear, and oil for backup) here in ERCOT. If anything happens to the gas supply in the future, the lights will go out. The common mode gas failure is not being studied in local reliability studies because there is not sufficient data from the gas suppliers to set up a model that will predict when gas supplies will be adequate and when they will be in short supply. Neither does the present market process here in ERCOT recognize that gas may be in short supply in the future or that prices might be very high. The gas industry is a big black box loaded with uncertainties. Neither do we account for the CO2 that natural gas plants produce and the environmental risk we are creating by building more and more gas plants. We are basically transforming our electric system to a single fuel dependent system with some energy from wind. I think this system design will ultimately leave us short and with higher electricity prices that if we continued to be diversified as we were in the 70s and 80s with new coal and nuclear plants. All the old guard is now gone and the young folks are weak at planning for a reliable system.
@Cyril R.
Your response seems to be yes, indeed, the point of the exercise is to make people stop investing in renewable energy, so that the money will flow to nuclear instead.
I would be interested to hear if Barry Brook agrees with that.
That position would be true if stopping renewable energy would add more nuclear generation than it would lose carbon free renewable generation.
That in turn does not seem plausible to me. Opposition to nuclear power is rooted in misguided beliefs about the dangers of radiation. Taking out renewable energy is neither necessary nor sufficient to promote nuclear energy.
Following.
Barry Brook — Fine, but for the continued use of nuclear ‘fuel’. Fissiles and fissionables are not fuels in the sense of being oxidized to produce energy in the form of heat. I propose calling those materials excallagants which are then excallagated in a nuclear reactor to produce energy in the form of heat.
I realize the term is not in common usage [I coined it the other day from a classical Greek word meaning ‘complete chage’.] Using it avoids any source of confusion on the part of those who understand little of the nuclear cycle. Examples: excallagant rods, partially excallagated rods in an excallagated rod pool.
I’ll be happy enough to switch to some other (technically defined) term; just not the sloppy use of ‘fuel’, please.
NREL has a number of studies on levelized costs and performance of concentrated solar thermal (for baseload generation), beyond the 2003 and 2004 papers you cite.
2008 Solar Technologies Market Report (2010).
Draws on REN21 Study Global Status Reports (2008): “the range of LCOE is approximately $0.12 – $0.18 per kWh for parabolic trough CSP, not including government incentives” (p. 50). Resource potential is assessed by Mehos and Kearney 2007, and Andraka 2008. Cost trend analysis suggests significant downward trend in costs over time. With storage added to the mix: capital expenses increase, but “the LCOE will most likely decrease because of the increased capacity factor and greater utilization of the power block” (74).
The Value of Concentrating Solar Power and Thermal Energy Storage (2010)
Looks at value of CSP in relation to size of solar field, optimization period, price and solar forecasting, ancillary service sales, capacity value, and dry cooling of CSP plant. Report specifically looks at cost competitiveness of TES component, and return on investment within a single year.
Sensitivity of Concentrating Solar Power Trough Performance, Cost and Financing with Solar Advisor Model (2008).
Use of Sandia National Lab Solar Advisor Model (SAM) for CSP. Shows sample results of LCOE in range of 14.51 cents/kWh for standard 100MW parabolic trough CSP plant with six hours of storage, and highlights impacts of important system variables to overall costs (solar field, engineering, procurement, and construction costs, and fixed O&M). Other models and analysis tools at NREL, and results for parabolic trough (TroughNet).
“Economic, Energy, and Environmental Benefits of Concentrating Solar Power in California” (2006).
“The CSP plants have nominal levelized costs in the range of $103 per MWh to $157 per MWh with the 30 percent ITC [investment tax credit] and $115 per MWh to $176 per MWh with the permanent 10 percent ITC. This is competitive with the 2007 simple cycle combustion turbine with a levelized cost of $168 per MWh (using the MPR natural gas price of $6.40/MMBtu escalated at 2.5 percent).” Comparative results are heavily impacted by capacity factors, learning curves, gas prices, optimization period (time of delivery of CSP energy) and more. Study also looks at projected greater return on economic activity and employment with CPS (compared to natural gas development). “With technology advancements, improvements to CSP construction efficiency, and with higher gas prices consistent with 2015 MPR projects, CSP becomes competitive with combined cycle power generation ($115 per MWh vs. $119 per MWh, even with the permanent 10 percent ITC)” (p. 8-2, emphasis added).
Beyond NREL, one can also look at $62 million R&D investment grants at DOE over 5 years for CSP. Pratt & Whitney (one of 13 recipients) lists as its solar program goal: “to make CSP cost competitive by demonstrating larger receiver design, enabling economy of plant scale to reduce LCOE. A power tower of 100 MWe or more can achieve under 7 cents/kWh with storage (12-17 hours) by 2020.” Other projects target storage costs at less than $15/kWhth, and lower costs on fabrication and collector technologies.
Based on this newer research, it’s unlikely that the high end of leveled costs for CSP cited in your paper ($169-246/MWh) is likely to remain that high for very long, or may have been based on faulty (or “out-dated”) assumptions in the first place?
It is simple Karl. We can’t control private investment much but when governments give away 100 billion in subsidies that is something ‘we’, if you will, have control over (after all that is the point of subsidies – pushing things in the right direction).
I see the root causes as fear, ignorance and supersition (to quote NNadir). This causes the totally fact-less and deluded support for ‘renewable’ energy and lack of investment and more generally a bad karma towards nuclear power.
Solar and wind in Germany can’t replace coal. Not even if they cost almost nothing. They are marginal. Nuclear can replace coal. It’s not marginal. This is an important message that isn’t coming through.
Just to be clear though I don’t suggest we ‘take out renewable energy’. I want people to come up with a plan that adds up, to quote another exellent energy thinker.
The reality is that Greenpeace and other quasi science (bodies) (deleted pejoratives)are frustrating nuclear development and one of their excuses is that solar and wind can take over. They even have faulty analysis (but with a vibe of soundness to it) to supposedly support that. And they’re in deep in policy making, they’ve even (influenced)(pejorative deleted) leading climate science groups.
The reality is that we don’t have a plan that adds up, and we are making it worse by fooling ourselves that we do.
I’m very pessimistic that we’ll deal with the problems. Nobody in charge is interested in solving them. We’ll just continue on business as usual for several more decades at least.
This TCASE 14 seems to be a remarkable turnaround from TCASE 13 where several fossil fuel technologies with CCS got the nod as Fit For Service.In 14 CCS is beginning to be seen for what it is,a smokescreen put down by the fossil fuel industry and its political supporters.
Now we have solar thermal with heat storage and gas backup being touted as Fit For Service.No mention of CCS for the gas backup,thankfully.Is this an attempt to placate the renewables enthusiasts? I don’t see this technology as a goer either for the following reasons –
(1) It still uses fossil fuels for electricity generation.I thought the objective was to remove fossil fuels from electricity generation – silly me.
(2) Solar thermal with heat storage is not a proven technology on any meaningful scale.
(3) It requires massive investment in collectors,storage and power transmission and after all this it still needs backup to deliver electricity 24/7 which is what is obviously required in anything but a third world society.
BNC is ostensibly a climate blog and it is well past time to take off the blinkers and the dunces cap and admit that we do not have the time or resources to build all sorts of highly questionable schemes so that we can luxuriate in denying that nuclear is the ONLY currently available technology which is capable of quickly making a huge dent in carbon emissions.
Podargus, on 18 August 2011 at 4:20 AM — Solar thermal with storage works fine so long as the location is never cloudy. There are a few places like that.
The main objection, as TCASE 14 clearly illustrates, is that it is much more expen$ive. Further, being a highly mature technology, the prices will not decline much if at all.
Karl-Friedrich Lenz,on 17 August 2011 at 10:31PM
“What is the point of this exercise? Stop all deployment of renewable energy and invest everything in nuclear?”
YES,with the rider that solar PV and possibly solar thermal does have niche applications but NOT in base load generation.
If Germany continues on its present course it will find this out the hard way,as in the past.
Podargus, on 18 August 2011 at 4:30 AM — I agree, based on my own studies of these issues.
A bit more on topic, NREL has a levelised cost calculator that allows you to find out yourself what the cost per kWh of an energy project is.
http://www.nrel.gov/analysis/tech_lcoe.html
This is great since you can get a feel on the effects of capital costs, capacity factor and the interest rate. So you can judge yourself whether a project makes sense rather than be boggled by the many differing estimates that we see in all sorts of reports. For example you’ll see solar advocates use very low figures for solar and higher figures for nuclear, and will see that this is simply wrong by trying to get their costs and see what interest rate etc. is needed to get that.
The calculator is pretty much self-explanatory.
The Greens evidently think we can bypass gas in the road to all-renewables
http://www.theaustralian.com.au/news/opinion/so-much-for-greening-with-gas/story-e6frg71x-1226116974834
Treasury modelling says Australia’s ‘gas fired energy’ will increase over 200% by 2050. That won’t be the case in SA, Vic and Tas who won’t have any gas well before 2050.
Elsewhere Federal energy minister Martin Ferguson says gas is a transition fuel. Transition to what? The leading CCGT + CCS project at Peterhead in Scotland needs a lot of money which is not forthcoming. That technology may never go anywhere with the LCOE figures being somewhat speculative.
Barry – thanks for this analysis. I’m wondering though… how do carbon capture and storage (CCS) power plants get into the ‘fit for service’ category? My impression is that, aside from a few rebranded/greenwashed enhanced oil recovery projects that inject CO2 into oil reservoirs, there aren’t yet any operating utility scale CCS projects. There have been announcements but no action. For example, The European CCS Demonstration Project Network says
Does the fit-for-service judgment come out of the studies analyzed in TCASE 13 and here? If so, how do they justify the assertion?
@Podargus, on 18 August 2011 at 4:20 AM: I absolutely agree that it’s foolish and wasteful to build things that won’t help. I include CCS systems as presently conceived in that list. We’d build equipment and infrastructure that has only one purpose, that doesn’t reduce the carbon intensity of the power plant to zero, that reduces the net energy produced by the power plant, and that will have no use after we convert to zero carbon emission energy sources.
with regard to Podargus, on 18 August 2011 at 4:20 AM
Solar thermal with heat storage is not a proven technology on any meaningful scale
I’d say it’s close. Gemasolar in Spain (20MW) did a 24hr run, including overnight – in midsummer, of course, but it’s a positive achievement.
http://www.physorg.com/news/2011-07-gemasolar-solar-thermal-power-hours.html
Cost of the plant was around $200 million I think, target capacity factor over 60%.
Joffan,
The LCOE for Gemasolar is about $450/MWh, or about ten times the cost of conventional baseload power.
Being able to run 24 hours in summer in Spain is nowhere near to being baseload capable. It needs to be able to run throughout times of heavy overcast conditions in winter. Such conditions often last for several days. To be baseload capable solar thermal must be able to handle these conditions and keep generating at full power. The cost of storage increases exponentially.
I guess I should respond to some of the questions on the study. Note that this was a single study on baseload generators. TCASE 13 looked at the mitigation part of the study. TCASE 14 looks at the costs part.
@ Zvyozdochka
Q1 – Our paper was accepted in October 2010, before the DOE 2011 report was released.
Q2 – EGS was considered as meeting the FFS criteria but reliable cost assessments were very difficult to find. I accept that the CCS cost estimates we had may also prove to be understated.
Q3 – I don’t believe LCOE calculations include site reuse capability. It would be very site specific if it did.
Q4 – of course.
@ David Benson
Wikipedia definition: “Fuel is any material that stores energy that can later be extracted to perform mechanical work in a controlled manner.”
Sounds vague enough to include U and Th.
@ Andrew Jaremko
Definition of FFS: For a technology to be considered fit-for-service (FFS) as a baseload generator it needs to be scalable, dispatchable without large storage and have a reliable fuel supply, low (L) or moderate (M) emissions intensity and a high capacity factor.
CCS meets all these criteria. I agree it is yet to be proven at commercial scale but that wasn’t in our criteria for FFS.
David B. Benson, on 18 August 2011 at 4:25 AM — are you saying that solar thermal with storage is a highly mature solution? I would have said that Gemasolar was pretty close to FOAK in terms of a solar thermal plant that can operate 24 hours a day.
I know conditions have to be close to perfect for this to be possible, but I think there is quite a bit of headroom for this configuration to improve. Getting to parity with FF-powered sources seems unlikely, but I don’t think that what is in play now is necessarily the apex of this technology.
Martin Nicholson, on 18 August 2011 at 9:58 AM — I cannot agree; by that definition a flywheel is “fuel”, complete nonsense.
————
FUEL — 1a : a material used to produce heat or power by burning
Origin of FUEL
Middle English fewel, from Anglo-French fuail, feuaile, from Vulgar Latin *focalia, from Latin focus hearth
First Known Use: 13th century
———-
I agree that the early developers of atomic energy picked up this term, probably not realizing the confusion it has later engendered in nonspecialists. I’m advocating a precise term which will not mislead.
@Cyril R.
Actually, the case for subsidies requires explaining that costs at market prices are too high. Feed-in tariffs are needed only because and only as long as some source of renewable energy can’t compete on price yet.
That of course means that you can’t redirect subsidies from renewable energy to nuclear, since nuclear does not need them in the first place.
As a basis for setting the feed-in tariff for next year on in legislation this summer, the German government has published estimates on costs as well. They are much lower than those published a couple of years ago by the US Energy Administration cited in the post above:
2011 German government report: is.gd/PWS3N8
Their estimate is 7 to 10 cent per Kw/h (= 70 to 100 dollars per Mw/h) for onshore wind (page 111) and 21 cent per Kw/H (210 dollars per Mw/h) for large-scale photovoltaic solar (page 130).
They also note that as a consequence of the feed-in tariffs solar photovoltaic costs have been reduced from 14.000 Euro per kw installed in 1990 to less than 3.000 today (more than 80 percent reduction), and they expect another 50% reduction in the next years (page 150).
Karl-Friedrich Lenz, on 18 August 2011 at 10:12 AM — Correction: the unit of energy required is kilowatt-hour, best abbreviated kWh. Your kW/h is a rate of change of power:
http://en.wikipedia.org/wiki/Watt#Confusion_of_watts.2C_watt-hours.2C_and_watts_per_hour
MickH, on 18 August 2011 at 10:06 AM — The scaling up to longer term storage requires no particularly large engineering advances; the thermal storage units have been in use for other purposes for a long time. So while continued progress can surely be made the cost of solar thermal with stroage is most likely to decline appreciably.
David B. Benson, on 18 August 2011 at 10:58 AM — true, in terms of scaling up that particular solution. But many other configurations could be tried (and some of them are in development atm) – fluoride salts at higher temps instead of nitrates, 1 tank storage instead of 2, super-critical CO2 Brayton cycle instead of Rankine, and (though this might be wishful thinking) a decline in the cost of manufacturing the array mirrors.
Every single one could be a dead end, but I’d be surprised. And I agree that solar thermal with heat storage is unlikely to ever reach @Peter Lang’s target of matching the current cost (or even the carbon-taxed cost) of FF power.
MickH, on 18 August 2011 at 11:30 AM — Somebody more in the know than I projected future costs for all these. The conclusion was that around US$0.20/kWh was about the best obtainable so unlikely to ever compete with cyclable NPPs.
K-F L is the reduction in PV costs attributable to German feed-in tariffs or China ramping up production? This was touched on in a recent documentary shown on Australian television
http://www.sbs.com.au/documentary/program/powersurge/about/synopsis
The question is whether China would have gotten into the market so heavily if Western countries had not such paid generous subsidies to PV buyers. If they’d done so regardless Germany could be claiming false credit.
K-F Lenz, I don’t intend to imply that we should focus on one solution, i.e. nuclear power. We need to use all the rational options at our disposal to eliminate our dependence on fossils fuels in short time frame and over a global scope. There will be a role for wind, solar, hydro, geothermal, wave and so on. I have no strong inherent objections to these technologies – they have benefits and disadvantages, just like all other energy technologies, including fossil fuels of various kinds and nuclear fission. Perhaps the biggest benefit of renewables is that the majority of the public apparently like them and think they are safe. Fine – this is a selling point as it gets a segment of the community ‘on board’. Others hate wind turbines or large dams, for instance – they can be catered for by supporting nuclear power.
However, I am strongly persuaded that cost, reliability and environmental footprint matters, and on this point, non-hydro renewables, especially the technosolar options, are currently losers – a bad investment. We need to be offering smart investments, if governments and utilities are going to make serious movements to build something other than a coal- or gas-fired power plant to replace an old unit when it is retired. TCASE 14 shows that nuclear fission energy is a winner – a smart investment – from a cost perspective. If people don’t care about costs and prefer other benefits that they perceive to get from things like solar PV, then fine, let them go ahead and build them and pay the cost. But society, overall, must bear the majority of infrastructure costs and we should not be wasting tax payer’s money on bad investments. The problem of fossil fuel replacement is too large, and too urgent, to play around with gimmicks when we know what we need to be focusing on.
If costs for renewables and energy storage continue to come down, and in the future they can compete with fossil fuels and nuclear on a level playing field, then that’ll be great too. The cost-benefit trade off will tip in their balance. Right now, the scales don’t tip that way, however, and it behooves people like us to communicate that fact, clearly, rationally, and with the best available evidence.
Solar thermal costs have a long way to go. The new Gemasolar plant in Spain is typical, as this post summarises: 20MW Gemasolar Plant: Elegant, But Pricey
To quote:
That is $33 billion per GWe average. Even at $6-8 billion per GWe for new nuclear in some countries (FOAK costs), it’s a steal by comparison.
This piece on “The Conversation” is appropriate to this thread:
http://theconversation.edu.au/solar-will-force-coal-and-nuclear-out-of-the-energy-business-2557
His figures don’t add up and you “techo” folks probably could put him straight on this topic.
Ms.Perps, in the article you link to, that breathless sort of triumphant optimism of ‘what will happen soon!’, coupled with a blasé attitude that permits the sweeping aside of the actual facts, is typical of newspapers puff pieces. What is staggering is that it was written by an academic.
Blakers is a renowned PV-phile.
IMO the article in The Conversation is too sloppy to merit inclusion in an educational forum. Everything it says should be referenced. Since State feed-in tariffs are undergoing frequent changes we need to see what current rates are and whether they are net-metered or grid export prices. That’s just to verify the claim PV is already cheaper than the grid in some States. Next question; we’ll have a carbon price why do we even need subsidies? Note today’s Crikey says RE is making grid prices $90/Mwh or 9c/kwh higher than necessary. A curious omission from the article.
Then the author needs to justify the amazing claim of nearly global grid parity for PV by 2015. On what basis? Ditto the claim on the need for just one day’s pumped hydro, even then a small amount. Note an unusually large chunk of the article is dedicated to dissing nuclear. How about equal space on CCS or geothermal? Not good enough.
@John Newlands
That is difficult to know. Anyway, the feed-in tariffs have provided a large market, and large markets lead to mass production.
@Barry Brook
I am pleased to hear that you don’t object very strongly to renewable energy. However, I am not quite convinced of the “bad investment” part.
That depends all on the question “bad compared to what”. The costs of feed-in tariffs are a bargain when weighted against the costs of having runaway global meltdown.
@ Ms. Perps et al.
Looks like this BS is doing the rounds today: http://www.smh.com.au/environment/energy-smart/solar-energy-cost-hits-par-with-coal-fuel-20110817-1iybc.html
An interesting quote from the SMH article though:
This suggests solar PV is not viable on a large scale without large and continuous subsidies – and hence, is completely unsustainable.
Karl, it does not matter that PV is getting cheaper. It is a marginal energy source, uncontrollable and not there 90% of the time in Germany. Germany needs a surprisingly constant supply of electricity, around 70 to 80% of the time. The implication is with cheap solar in Germany you build a bunch of solar, maybe 15-20%, and you burn fossil for the other 85-90% of your demand.
That is called fossil lock in. Paying 100 billion bucks in various FITs for a fossil lock in is not a good investment Karl.
Nuclear is likely somewhat more expensive than cheapest dirtiest coal so it definitely could be sped up by subsidies. For 2 dollars per Watt subsidy on a nuclear plant you’d get 50 GWe of highly profitable nuclear plants which is sufficient for almost all current fossil served demand in Germany. You’d get 40-45 GWe average electric flow. You could close all coal plants – forever. By contrast 50 GWe peak of PV installed capacity would cost a lot more especially with FIT that everyone touts for reasons unclear to me (it is one of the most expensive and inefficient, though effective subsidy methods) and it would get you only 5 GWe of average electric flow. That’s why I say marginal. If PV is to make a big contribution it needs to be not only cheap, it also needs cheap storage and that is not in the pipeline in any development right now. So the PV advocates rely on a deus ex machina on energy storage, or hand-wave the problem of storage away with qualitative statements (“smart grids yeah”).
Until recently, I have been arguing that renewable energy has its place “in the mix” on the smallest scale: homesteads, rural communities, etc.
I had been sold when a station (ranch) manager had proudly showed me over his wind and solar system. Lead acid storage supplied the homestead lighting and electronics. A larger equivalent of storage consisted of intermittent pumping of dam water out to the livestock troughs through many kilometres of poly-pipe.
Two weeks back, we met again. He has gone back to diesel generation. Suppliers have soaked up all the government subsidy, he said, and renewable energy is now too expensive for him and his kind.
If renewable energy can start small, it can’t take over the world.
Barry,
Re the ENC blog extract you posted that in turn quotes the Daily Mail. I wouldn’t trust anything from the Daily Mail as they also run articles promoting astrology as ‘true’. I would look to the Australian, despite its continuing War On Science (aka The Glass Jaw of the Nation), before I accepted any figures quoted in the Daily Mail.
Perhaps the ENC blogger just doesn’t know anything about the Dail Mail.
Yes, the benchmark LCOE you provide for Gemasolar (without citation) is very high. The NREL reference I provided above gives a much lower LCOE for the first CSP plants installed in 2009 (with six hours of storage), and a projected cost reduction of 22% by 2015.
With no carbon price … “A comparison of the levelized cost of energy (LCOE) revealed that the LCOE of $148 per MWh for the first CSP plants installed in 2009 is competitive with the simple cycle combustion turbine at an LCOE of $168 per MWh, assuming that the temporary 30 percent Investment Tax Credit is extended. The LCOE for the CSP plant is higher than the $104 per MWh LCOE of the combined cycle combustion turbine plant” … CSP plants installed in 2015 are projected to exhibit a delivered LCOE of $115/MWh [drops to $103/MWh with 30% ITC], compared with $168/MWh for the simple cycle combustion turbine and $104/MWh for combined cycle plants. At a natural gas price of about $8 per MMBtu, the LCOE of CSP and the combined cycle plants at 40 percent capacity factor are equal” (p. ES-2).
Gamasolar has been very successful at achieving high capacity values for a 24 hour CSP plant (@ 63.1%), but lets look at industry as a whole (and more recent cost reductions from mirror fabrication and lower design, materials, and O&M costs) to arrive at more likely baseline for future projections.
Additional support for lower cost estimates for CSP:
– You can’t get any more robust than the work of MIT engineer Alexander Slocum, and his paper “Concentrated solar power on demand” (in peer reviewed Solar Energy). Looking at a new type of tank construction for molten salt storage that could reduce equipment needs and increase durability, Slocum estimates LCOE for CSP prototype in range of $0.07-0.33/kWh with range determined by turbine capacity, size of mirror field, quality of resource, and reduction in equipment needs.
– DOE Report to Congress: “Assessment of Potential Impact of Concentrating Solar Power for Electricity Generation” (2007). Cites 2002 DOE report that suggests with $1.5 to 2 billion investment over 14 years, “CSP could provide hundreds of gigawatts of electricity at 5 to 6 cents/kWh without further subsidies while also providing economic, environmental, and security benefits” (iii). 2000 National Research Council report contrasts with these results, and Sargent and Lundy Report (2003) was commissioned to resolve the differences: “the cost of electricity from CSP was currently in the range of 10 to 12.6 cents/kWh, and costs could be reduced to 3.5 to 6.2 cents/kWh by 2020 without new research breakthroughs” (iv).
– Peer Reviewed Article: “Dish Stirling technology: A 100 MW solar power plant using hydrogen for Algeria” (2010): projects LCOE as low as 11.55 cents/kWh depending on site location (as high as 23.55 cents/kWh). These beat cost estimates of Ecostar European Concentrated Solar Thermal Road-Mapping study (2004), i.e., 15 – 20 cents/kWh for current technology, and match 2000 projections for CSP cost estimates in India.
– 2011 Report from GreenTech Media (available for purchase): “In the next decade, the research firm [GTM] projects CSP plants will be generating electricity in the $0.10 to $0.12 per kWh range and PV will be producing electricity in the $0.07 to $0.08 kWh range [more cost effective than natural gas]. (On the flip side, CSP technologies can offer storage capabilities and hybrid natural gas components, providing value that PV can’t necessarily deliver” (report summary by Stephen Lacey, editor Renewable Energy World).
One can also look to projected new plant construction costs, feasibility studies, and power purchase agreements to find a balance of LCOE range estimates for current CSP (far less than unsupported $450/MWh number provided by Lang, or high end projections cited in Barry Brook, et. al., article).
– Interview with Kevin Smith, CEO of California start-up SolarReserve: wholesale power purchase agreement with NV Energy for Crescent Dunes 110 MW project (with 12 hour storage) in Tonopah, Nevada, at 13.5 cents/kWh, “we certainly can compete head-to-head directly with nuclear.”
– eSolar and B&W feasibility study, and SkyFuel Award Review: research to meet DOE funding criteria of 100 MW plant, 75% capacity factor, and LCOE that is competitive with baseload fossil fuel plant ($0.08 – 0.09 cents/kWh by 2020).
– PG&E, Southern California Edison, and San Diego Gas and Electric all have power purchase agreements for CSP plants in S. California, but there is little information on disclosed amounts.
Agreed!!
Roger Clifton, on 18 August 2011 at 7:31 PM
There is something odd about your station managers story.Once the wind and solar system plus battery back up is installed (and paid for) there would be only a need to use the existing diesel generator for prolonged cloudy conditions or for heavy current draw like large electric motors.
I believe that the federal government subsidy for renewable energy applications for sites remote from the grid is paid upfront to reduce the installation cost so that could not be a factor.So the claim that suppliers have soaked up the government subsidy doesn’t make sense.
It is always dangerous to take an individual case and extend it to others and there is more to this story than meets the eye.
Going back to diesel generation while scrapping a wind and solar system would be far more expensive to run and becoming more so.
While I believe that nuclear is the only viable solution for clean base load power I think that solar,in particular, has a great future in areas where grid connection is not practical.
Podargus, on 19 August 2011 at 6:27 AM said:
Solar system plus battery back up is installed (and paid for) there would be only a need to use the existing diesel generator for prolonged cloudy conditions or for heavy current draw like large electric motors.
Depth of discharge and cycling can have quite negative impacts on battery life.
http://pvcdrom.pveducation.org/BATTERY/charlead.htm
@CyrilK
You said it does not matter that solar energy is getting cheaper.
It does. Actually, that is exactly the point the post above has addressed.
The other problem is the fact that the sun does not shine all the time. That is mitigated (for Germany) by the fact that about one half of renewable electricity produced in 2010 is from biomass and hydro. These work even when there is neither wind nor sun available.
http://is.gd/PqizSZ German government report on 2010 production.
It seems that the largest CSP project in the world – Blythe Phase 1 in California (500 MW) – is to be abandoned for PV. This brings the total capacity of proposed CSP projects in the US that have been replaced by PV to nearly 3 GW:
http://thinkprogress.org/romm/2011/08/18/298913/the-worlds-largest-planned-solar-plant-switching-csp-to-pv/?utm_source=feedburner&utm_medium=email&utm_campaign=Feed%3A+climateprogress%2FlCrX+%28Climate+Progress%29
Jeremy C:
Noted for reference. Prompted by this, I went to another source, the National Renewable Energy Laboratory page on Gemasolar. Based on this data (17 MWe peak, and calculating a 67% capacity factor), they give a capital cost of 230 million euros. Crunching the numbers, this gives $28 billion per GWe average, which is similar to the Daily Mail figure of $33 billion. Of course, we have to trust the projected performance data – if it doesn’t meet forecast specs, the cost will be higher. I can feel a new TCASE coming on…
The physical input to mass storage of PV generated electricity via lead-acid batteries is discussed here
http://www.theoildrum.com/node/8237#more
The author says there is little alternative for most night time electricity demand to be generated in real time i.e. at night. A follow up article asks whether PV can smelt silicon and so on to replicate itself.
Surely when millions of people want coffee and toast at 7 a.m. they could reach into an insulated box from when it was prepared the day before. We need more subsidies to make it happen.
EL @ 19 August 2011 at 2:07 AM
I calculated the LCOE for Gemasolar using the NREL calculator: http://www.nrel.gov/analysis/tech_lcoe.html
And inputs:
Period (economic life) = 20 years
Discount rate = 8.5%
Capital cost = $23,225/kW
Capacity factor = 63%
Fixed O&M cost = $50/kW-yr
Variable O&M = $0.002/kWh
Heat rate = 0
Fuel cost = 0
LCOE = $450/MWh (approx)
Inputs were from:
http://www.nrel.gov/csp/solarpaces/project_detail.cfm/projectID=40
http://europa.eu/rapid/pressReleasesAction.do?reference=BEI/09/224&type=HTML
http://www.solarpaces.org/Tasks/Task1/Task%20I.pdf
The renewable energy advocates have been making these sorts of projections since the 1980’s. They are almost always wrong. For example the NEEDS analysis projected that LCOE of solar thermal would decrease 30% from 2007 to 2010. However, during that period the LCOE went up by 30%, not down.
This is not a fair comparison. Firstly, OCGTs are peaking units that provide power on demand. They are dispatchable. Solar is not. The OCGT delivers very high value power, the solar plants deliver low value power “take it whether you want it or not” type power.
Secondly, it is not a fair comparison to calculate the LCOE after the subsidies have been included. For a fair comparison LCOE must be calculated on the cost of the plant excluding all subsidies.
If you believe that, you’d believe anything.
Just to finish off, the LCOE for a new, state of the art, solar PV power station at Windora Queensland is $1,445/MWh. The cost per tonne CO2 avoided is $6,000 per tonne avoided.
http://ecogeneration.com.au/news/windorah_solar_farm/011780/
Further to the above, I used the 63% capacity factor rather than 67% because the plant is now quoted as 19.9MW capacity where as previously it was quoted as 17MW. However, the expecred annual generation is still 110,000MWh. So the new capacity factor is 63%. I expect this will not be achieved over 20 years. It is inevitable there will be breakdowns and various problems as there always are with such plants.
Karl, biomass and hydro in Germany can’t expand to replace coal and natural gas fired generation. There’s not enough of it for Germany. So your point that an energy source that is not there 90% of the time is mitigated by the fact that there is a small amount of hydro and biomass is nonsense.
This borders the absurd. If hydro and biomass are good energy sources they will work much better with nuclear, as nuclear is there 80 to 90% of the time. The fact that hydro and biomass might be good energy sources is completely seperate and independant from what you do with the rest of your energy supply. PV on the grid in Germany sucks. Keep the biomass and hydro, don’t build the PV.
Please just run the numbers. Grid connected PV doesn’t add up, certainly not in Germany. Nuclear does. With some hydro and biomass, a nuclear grid is a 90% solution.
Karl, “You said it does not matter that solar energy is getting cheaper.
It does. Actually, that is exactly the point the post above has addressed.”
The point is that PV prices are not that relevant for getting rid of fossil on the grid. For that to matter you also need cheap storage; that could make PV non-marginal. That’s simply not in the pipeline, at all. No company has a storage scheme cheap enough, there are only press releases and theoretical work. Deus ex machina. And as others have suggested, with nuclear you could also use that storage which will be cheaper since you need less of it (due to constant output and little multi day variability).
Here you can get an idea of the scale of energy storage we’re talking about:
http://www.theoildrum.com/node/8237
As you can see we’re not going to do this. In stead, with cheap PV what we will do is build a bunch of PV at best close to summer peak demand in capacity, which will be not there 90% of the time in Germany, so we’ll be seeing something like 20% solar 80% natural gas. It would be silly to talk about that natural gas as backup for solar – its a natural gas grid with some solar to make people feel good.
Maybe its just me, but I don’t like this scenario. Its fossil fuel greenwashing & lock in. As long as we keep fooling ourselves that marginal energy sources can supply us and solve the fossil addiction, we’re not moving ahead. That’s why we want people to come up with a plan that works. First run the numbers then make the commitment. I believe this is an important part of the reasoning behind the TCASE series here.
Some of these numbers are candidates for revision? May 24, 2011, Torresol Energy press release for Gemasolar plant reports 19.9 MW turbine and EUR 171 financing ($239 million in 2010 USD dollars). This is confirmed in Platts Global Power Report via Lexis-Nexis (May 26, 2011). Capacity factor is 74% (technical director Torresol and NREL). We’ll stick with 20 years, but typical life cycle for CSP molten salt plant is 30-40 years (here and here).
Period = 20 years (some recommend 30 years for CSP)
Discount rate = 8.5% (high amount, FEMP recommends 4% for energy projects)
Capital cost = $12,010/kW
capacity factor = 74%
Fixed O&M = $50/kW-yr
Variable O&M = $0.002/kWh
Heat rate = 0
Fuel cost = 0
Gemasolar LCOE (via NREL calculator) = $204/MWh (approx.)
4% is way too low. I work for a big engineering firm myself and that 4% would put us out of business forever. You need to get some sort of funding outside of the bank’s loan (debt), otherwise the bank won’t get you the money. Bank might get us 6-7% and the ‘other’ investment which is basically private investors (stockholders etc – called equity) will demand way over 10%. Energy projects are very risky things. There’s risk of delays that mean you don’t get your money when you thought you would, and there’s risks of market dynamics that make your plant output redundant. Theres also risks of new technology: it might break down all the time which really hurts the business case; you need to buy expensive replacement power while not making any money at all, in fact you need expensive repairs!
8.5 percent is okay for an average rate between the bank’s portion of the loan and the private investment. If you are a credible company and your techs are trusted then you might get more money from the bank at that lower interest rate. Definitely you won’t get it at 4%. You might get it at 6% and if you get 70% of your money this way and 30% of your money privately at 12% then your weighted average discount rate is 7.8 percent. But you are lucky to get 70% from the bank, these days the banks are not taking that kind of risks unless you are a very credible firm and are building a well known energy technology. But those things are not the case for new CSP startups, they are not credible at all (sorry!) and their technologies are mostly unproven especially at this scale. I would not be surprised if the average discount rate these companies get for their projects is over 12%. It is only profitable because of massive subsidies from governments such as Spain (like 5-10x wholesale electricity market prices!!). If your subsidy drops out because Spain has run out of money (this is happening right now) then your project is dead and investors lose much of their money.
And one more thing, EL, the cost of Gemasolar is not 171 million euros, the 171 milion euro figure refers to a financing portion that they have managed to secure. There are other financing packadges required to get your project done (almost never just 1 private investor). Total cost seems to be 260 million UK pounds according to the Energy Collective.
http://theenergycollective.com/nathan-wilson/58791/20mw-gemasolar-plant-elegant-pricey
Cyril R.
You are right to a certain extent. The projections for 2020 (with renewable hitting 38% of all consumption) show that hydro and biomass will be down to one third of all renewable, from one half in 2010, since they have less potential for expansion.
That is still a significant part that is completely flexible.
But you are right as well that storage will be important. We will see if it is impossible to do, as you seem to think.
One interesting way to do this has been implemented in 1978 as a 321 MW storage project for a nuclear plant (storing at night when prices are low and putting the energy back in the grid at higher daytime prices). It works by compressing air.
http://de.wikipedia.org/wiki/Kraftwerk_Huntorf
Yes, with nuclear storage works very well since you need only several hours to meet almost all demand this way. That’s a high turnover (once a day) with a low amount of storage (cost) that is very nice.
Ironically most storage schemes were developed to use baseload power such as nuclear or coal and store it for peak times to save expensive peaker generation.
If the Germans can get 15% hydro and biomass they can use 85% nuclear which will be a good fit especially with plugin hybrids.
The problem with solar in Germany is that its almost nothing in winter, when power demand is highest. They could overbuild solar but that is expensive since you have to dump the excess during summer (just when the sun is more productive).
I don’t think storage is impossible. It might be, in the case of batteries, if we can’t get enough lead, lithium etc. More important I think is that it will cost so much that we will prefer to instead burn fossil whenever its not sunny (which is 90% of the time in Germany, ie 1000 solar Watts give an average of 100 Watt flow).
The Germans will have to find out the hard way that their plans don’t add up. They are very stubborn and don’t care about basic energy analysis. They just want to feel good and prefer to do this with cool and sexy solar panels.
I think Peter Lang above makes a good point about ‘storage’. There is storage and then there is ‘storage’. Pumped hydro, in the few places this can be developed, is often has enough volume to run for days without replenishment. As I’ve noted here, the Helms Pump Storage facility can run for almost 2 weeks straight, 24/7 at full 1200 MWs generation. It was built in conjunction with Diablo Canyon Nuclear Power.
But that is an exception. Peter points to the only “24 hour” CSP plant in Spain, for “19MWs” at best. How will the costs “come down” in this insanely high priced power? And then to make it last, say, 72 hours? Ah, expensive, and polluting diesel back up or natural gas back up.
I think what you really see in terms of non-nuclear low carbon energy is “all about wind” and it’s wind that has really taken off in Europe. This is what is parsed and what needs further discussion. Despite the money being poured down the drain on solar projects, and as show by the statistics and real life operating experience, wind is where it’s ‘at’ in terms where the energy is coming from and where the money is really going. And all the many problems associated with that.
As for “policy”, we need to put money into energy generation. I know Peter’s libertarian principals don’t allow for much in terms of subsidies, but in the US, the really big projects need lots of gov’t back up (no hydro unit has ever been built with out total Federal monies). I’m for society saying “yes, let’s invest in nuclear or this or that technology and get it deployed. That’s what modern civilized societies do. If we let the false, a-historical paradigm of the “free market” decided, we’ll all be living with kerosene lamps and horses for transportation.
Regarding cost of Gemasolar project:
Jeremy C made comment above that UK’s Daily Mail might not be a most trusted source (for astrology no less), and this seems to be the main source for the Energy Collective blog post by Nathan Wilson.
We have many numbers floating around here (converting for current dollar values):
Daily Mail
– £260million ($299 EUR) ($429 USD)
NREL Project Site:
– $230 million EUR (£199) ($329 USD)
Torresol Press Release (on financing from multiple sources including Banco Popular, Banesto, ICO and the European Investment Bank)
– $171 million EUR (£148) ($244 USD)
Seems like someone needs to nail down Gemasolar total construction cost before making final claims about LCOE (particularly when out of range of $0.10 – $0.18 cents/kWh (USD) acknowledged by industry analysis as “best case” benchmark LCOE for current CSP, here and here).
If Gemasolar is really that important to argument: I’d take NREL number ($329 USD, an inflated number in 2011 dollars), 4% discount rate for power projects (recommended by FEMP for LCOE calculations), 30 year plant life, 63% capacity factor (lower amount), and arrive at LOCE of $18.3 kWh (high end of range suggested by other sources above).
Worth including here … key study by California Energy Commission looking at comparative costs of 21 central station generating technologies (in service in 2009 and 2018): “6 gas-fired, 13 renewable, nuclear, and coal-integrated gasification combined cycle. All levelized costs are developed using the Energy Commission’s Cost of Generation Model” (xiii). Included in report are assessments for nuclear (Westinghouse AP1000 in service in 2018) and CSP Parabolic Trough (considered in TCASE 14). Projections made on basis of Merchant Owned, Investor Owned (IOU), and Publicly-Owned (POU) utilities.
Among the key findings:
– Price trends: Costs for nuclear “rise beyond competitive range” over time, but costs for wind, coal-integrated gasification combined cycle (coal-IGC), and solar (CSP Parabolic Trough and PV Single Axis) trend downward. “The other technologies show no or very little cost improvement [over time]” (p. 17).
– New Nuclear (40 year book life) is more expensive than CSP Parabolic Trough and PV Single Axis in 2018 (for Merchant Plants), p. 20.
– “Solar photovoltaic, which has shown dramatic cost change since 2007, is expected to show the most improvement of all the technologies, bringing its capital cost within range of the gas-fired combined cycle units” (17).
So where do I contact the FEMP about this? I’d like to ask them some money, at 4% interest, my company will take two million.
Discount rate is not the same as the project compound average interest rate (weighted average of debt+equity).
The 171 million euros is not from multiple sources, it is from one with many other journalists/sources quoting each other without question. Hardly a suprise. It’s quite obvious that this is a single round of financing, and it’s highly unusual for such 100 million plus projects to be financed with just one equity round…
New nuclear more expensive than CSP and PV? See the NREL calculator above – this is pure nonsense sold by interest groups. Find out yourself using the calculator. California gives hefty subsidies to solar but over-taxes nuclear. Even with that nuclear’s a better business case – and its actually non-marginal so its better for ‘society’ if you will. Meaning it can stand on its own without burning 80% natural gas.
Of course, the California Energy Commission is a commission in fantasy land, so nobody should be surprised to hear axes grind.
EL, on 20 August 2011 at 1:44 AM — Here is the final staff report from the California Energy Commission:
http://www.energy.ca.gov/2009publications/CEC-200-2009-017/CEC-200-2009-017-SF.PDF
I’m going to read this latest version.
Cyril R., on 20 August 2011 at 2:22 AM — The NREL simplified LCOE calculator is a bit crude.
If I have it correct, discount rate is a way to assess the future effect of inflation on a long term loan and opportunity cost of money (that money is “worth more” at beginning of the loan period compared to the end with rising inflation). Stated in another way, it is a way to assign the present value of a loan to future cash flows.
Federal Energy Management Program (FEMP) Executive Order 13123 (“Guidance on Life-Cycle Cost Analysis” and “Energy Price Indices and Discount Factors for Life-Cycle Cost Analysis – 2010“) provides instruction on how this is to be calculated for energy conservation, water conservation, and renewable energy projects:
“Subject to a ceiling of 10 percent and a floor of three percent the real discount rate shall be a 12 month average of the composite yields of all outstanding U.S. Treasury bonds neither due nor callable in less than ten years, as most recently reported by the Federal Reserve Board, adjusted to exclude estimated increases in the general level of prices consistent with projections of inflation in the most recent Economic Report of the President’s Council of Economic Advisors.”
Currently, on the basis of Price Index and Discount Factors for 2010, NREL LCOE calculator recommends a 4% nominal discount rate for energy projects, or 3% real for federal projects. If you want to use something higher, you’ll have to provide justification for this with respect to a higher rate of inflation or interest rate over specified period of loan.
David B. Benson, on 20 August 2011 at 2:48 AM said:
final staff report from the California Energy Commission:
Table 22 is interesting. It appears that the Westinghouse AP1000 was downrated for California to have a Capacity of 960 MW and utilization rate of 86%.
What matters is at what average percentage you can get the money. It does not matter that the economists use discounting to value macro-economic aspects. The rate at which you, the project owner, can get your money, is what determines the price you have to get per kWh to break even or to get a certain amount of profit from the project.
4% is way too low. No one will lend you money for 4%. Too risky for a single project.
harrywr2, on 20 August 2011 at 3:25 AM — Yes. I can only assume that they plugged in the operational history of the precisely 2 NPPs, both Gen II, in California.
Furthermore, in one table the O&M largely agreed with NREL’s recommended range and in anothr table waas cranked up to $147/kW-yr. (Maybe the latter is supposed to include fuel costs.?)
Anyway, several years ago E3 did a similar study for the State of California and arrived at a fairly outrageously high LCOE for NPPs. Neither study, even taking into account the sorry state of financing NPPs in the USA versus other countries, is in agrrement with build costs experienced elsewhere in the world — not even the overbudget/overdue first Areva EPR.
Furthermore, this report doesn’t even manage to correctly state matters for the GE H-frame CCGT. Since SCE has at least two in their natgas generation park, you would think at least that could be done correctly.
All told, it doesn’t appear to me that the California stake-holders are receiving the best advice.
David B. Benson – you mentioned you thought the NREL levelised cost calculator is a bit crude. I disagree, after over 12 years of professional experience on various projects for two different companies, doing all sorts of cost calcs, I’ve found out that the simpler models are more useful. Lots of companies use complicated models and they often end up being less succesful than the guy with the simple plan. The simpler the model the more difficult it is to screw up and the more robust it is. NREL’s online calculator has everything you need to know to get the levelised cost. Perhaps even more importantly, everyone can use it. That’s an important educational aspect.
Sure, you might have a model that is more complicated that shows you how much debt and equity you have, but the only practical implication for levelised cost is that this affects the average interest rate. So you are being more elegant by asking for the average interest rate on your total funding.
Areva’s first EPR, by the way, is pretty cheap actually, put them into the NREL calculator, and find out.
7.2 billion USD for 1.6 GWe is 4500 USD/kWe. 0.92 capacity factor (typical lifetime design target) 10% average interest rate (I see now that the NREL gives ‘discount rate’ which is the wrong term that might have caused some confusion…). Fixed operations and maintenance is 50 dollars per kWe-year, I don’t use BTU heat rate (silly victorian units) just be simple and use 2 cents (0.02) variable costs (includes waste dry storage and plant decommissioning). 40 years financing period.
http://www.nrel.gov/analysis/tech_lcoe.html
This gives me 6.5 US cents per kWh simple levelised cost. Not bad. Could be that they use a shorter financing period, in which case it will be more, but you get 2 cent per kWh electricity after the plant is paid for. : )
It seems that new NPP construction is proceeding at a more rapid rate than at least I realized. I know that Vogtle 3&4 were in the so-called pre-construction phase (before any reactor containment concrete pours), but I didn’t know that the Summer NPPs were in a similar stage:
http://www.world-nuclear-news.org/NN_Final_days_of_Summer_licensing_1908111.html
But more, TVA is finishing up building one and is restarting the buiding of another:
http://www.world-nuclear-news.org/NN_Decision_to_build_Bellefonte_1_1908111.html
So despite the financing woes, I’d say matters are not entirely hopeless here in the USA.
Cyril R., on 20 August 2011 at 4:55 AM — Thanks. The NREl calculation leaves out taxes and maybe other financial matters; for technology comparison I don’t think it matters.
As for the first Areva EPR, I don’t know what the payback period is, but in the USA 30 years is as long as one can obtain and the DoE load guarantee is for 10.8%, not less. Your estimate for O&M is probably too low for the USA; maybe US$60/kW-yr is obtainable.
I didn’t know about the variable O&M trick. Assuming your US$0.02/kWh is correct for fuel related costs, I obtain US$0.073/kWh. I suspect both of us are leaving something important out of the calculation since both your figure and mine are much below the so-called professional estimates.
David, it’s pretty bad here in the US. 4 plants and that’s really it. Everything else is ‘holding’, in reality and will be more viciously challenged by the antis.
There are still about 24 plants languishing in the NRC docket. I’m not holding my breadth, and neither should anyone. Nuclear’s development will be other countries besides Germany and US.
David B. Benson, on 20 August 2011 at 5:10 AM said:
It seems that new NPP construction is proceeding at a more rapid rate than at least I realized….so despite the financing woes, I’d say matters are not entirely hopeless here in the USA.
Things are moving along in Florida as well, it appears the Florida Utilities will be able to hang onto being able to bill out ‘construction financing costs’ as they occur rather then rolling them into the cost of the project.
http://www.sunshinestatenews.com/story/progress-energy-gets-small-rate-hike-big-nuclear-costs-ahead
Current US NRC licensing schedule –
http://www.nrc.gov/reactors/new-reactors/new-licensing-files/new-rx-licensing-app-legend.pdf
The US Southeast doesn’t have very good wind(Hurricanes don’t count) and despite Florida being called the ‘Sunshine State’ it’s actually fairly cloudy there.
David Walters, on 20 August 2011 at 7:05 AM — As I count it, 1 actually under construction (TVA), 4 in site preparation, 1 more rather definite to come as TVA is restarting a half finisihed unit, 2 more very likely in Florida. NRC is pushing the COLs through as fast as it knows how, but given the current low cost of natgas, who knows how many of those will actually be built.
I’m more optimistic about the future of NPPs in the USa than you appear to be. Eventually decision makers will begin to see that wind+olar displaces no coal burners.
David Walters, on 20 August 2011 at 7:05 AM said:
There are still about 24 plants languishing in the NRC docket. I’m not holding my breadth, and neither should anyone
Holding makes economic sense.
There are 3 risks reflected in financing rates
1) Regulatory – Voglte #3 will answer the regulatory risk question as to regulator risk related to the AP1000
2) Construction Risk – Again Vogle #3 and #4 will answer the question as to whether an AP1000 can be built on budget
3) Demand risk – it’s awfully hard to make a demand projection when you are in the middle of a recession without an ‘end date’.
EL,
I’ll stick with my LCOE figures for Gemasolar (approx $450/MWh)
First, your cost of “$239 million in 2010 USD dollars” is not the full cost. I gave you the references. Did you read them? Did you find errors in these documents that you can substantiate from an authoritative source. The references you gave are the proponents/owners marketing promotion and 2006 figures from NREL. I wouldn’t rely on them as authoritative.
Therefore, your cost per kW is not correct. It is too low. The figure I gave is the last total project cost figure I have seen; however, I expect it could have increased since as most projects do during construction and commissioning. If the capacity has been increased, as stated, I expect there was an extra cost. How much was it?
Second, what I’ve seen is that the expected generation per year has not changed. It is still 110GWh. Even if you say they have increased the size of the solar field and the storage capacity to give more energy per year, then I’d expect there has been a cost increase.
Furthermore, I would not rely on the renewable advocates’ projections. I think they are likely to be found to be optimistic, if we are cautious, we should probably discount their figures. So I expect the plant, as it is now, will not generate 110GWh on average for 20 years. I expect it will be much less. (are they relying on the extra power being provided by increased natural gas capacity?)
This raises the question: Did they rely heavily on the natural gas generation to allow the plant to generate for 24 hours? If so, it is being a bit dishonest to imply the solar plant achieved 24 hours full power generation as a solar thermal plant using its own energy storage.
Third, as CyrilR has pointed out the figure of 8.5% weighted average cost of capital (WACC) (or discount rate) is about right for mature proven plants like coal, gas and hydro. A much higher discount rates is needed for nuclear because of the investor risk premium demanded (thanks to 50 years of anti-nuke protesting, delays, disruption, early shut-downs, etc), and an even higher discount rate would be needed for solar. In fact the discount rate is not really relevant, because they would not be built no matter how high the discount rate if not for the government subsidies.
This EPRI report http://www.ret.gov.au/energy/Documents/AEGTC%202010.pdf compares LCOE for new entrant electricity generators in the Australian National Electricity Market. The WACC (or discount rate) used for the study is 8.4% (see Table 10-1, page 10-1)
Fourth, you argue that the expected life of the plant where is it economically viable (i.e. without any subsidies) should be more than 20 years. I disagree. But please produce the figures for any solar thermal plant with energy storage that has produced 63% capacity factor for longer than 20 years without any subsidy whatsoever; then we can begin a discussion. Until then, the economic life of renewable energy plants is not reality.
Until we have several years, preferably a decade, of operating performance and costs – and we understand what is the minimum output in worst case climatic conditions, we should take the projections as highly optimistic.
Therefore, until I see reliable figures based on actual performance and costs, I will continue to use the most authoritative and conservative figures available. For now I am persuaded the best figure for the LCOE of Gemasolar, a natural gas hybrid plant, is $450/MWh; i.e. about ten times the cost of conventional baselood power
David Walters, @ 20 August 2011 at 12:40 AM
You misunderstand my position. You say: “I’m for society saying “yes, let’s invest in nuclear or this or that technology and get it deployed.”
However, that route does not lead to nuclear. It does not lead to a practicable, economic solution. It leads to renewables – the emotionally attractive but totally uneconomic, non-solution. We’ve got 50 years of evidence to prove this and the evidence is getting stronger all the time (look at Germany now). Your solution is exactly the wrong solution. The better solution, as I‘ve explained many times is to remove the impediments to a freer market in energy, allow the market to operate and regulate it lightly but appropriately. Regulation will include incorporating externalities to the extent that is a net benefit to society.
Consistent with this approach, we could change the renewable energy targets to clean energy targets, and allow the market to choose the least cost option that achieves all the objectives we require.
Adding another government imposed, market distortion – a carbon price – is exactly the wrong policy. Carbon Pricing is the exact opposite of what I believe is the policy we need to implement to get us to low emissions energy (see this excellent comment by me that no one commented on : http://bravenewclimate.com/2011/08/09/ipcc-renewables-critique/#comment-133186 .
David Benson,
That’s not correct. You just have to decide whether you want to use before or after tax WACC and enter the appropriate value. The decision is not trivial. The weighted average cost of capital (WACC), which is what the NREL calculator calls “Discount Rate” can be before or after tax effects. The EPRI report uses before tax. http://www.ret.gov.au/energy/Documents/AEGTC%202010.pdf table 10-1, page 10-1. The economic assumptions are in Section 5.
The ACIL-Tasman report has an good explanation of WACC and the various methods of calculating it (its not trivial): http://www.aemo.com.au/planning/419-0035.pdf
See Section 2.2.2 “Discount Rate” and Section 2.4.2 “WACC for new entrants”.
The ACIL-Tasman report uses after-tax WACC.
I’d urge you to look at these if interested in WACC.
Peter Lang, on 20 August 2011 at 10:59 AM — Thank you.
Peter Lang, @ 20 August 2011 at 9:17 AM
I very much appreciated your comment, especially the sentence: “Regulation will include incorporating externalities to the extent that this is a net benefit to society.” On the extent to which Australia’s proposed carbon price meets this criterion, we probably differ, but not on the standard itself.
@Podargus, on 19 August at 6:27 AM argues that my informant’s claim that suppliers of renewables equipment have soaked up the government subsidy doesn’t make sense. After all, the subsidy is for first installations, not for maintenance.
I think the station manager was referring to the fact that parts for his ongoing maintenance were being bought off the same suppliers of first installations. With the ready cash supporting first installations, that they had increased their prices to match the cash available. If so, the installation subsidy would undermine long-term renewable operations.
Small installations inevitably have higher maintenance costs per capacity. Replacement of lightning-damaged electronics, bird-damaged blades, gypsum-smeared solar panels and so on will always require at least one skilled worker. Protection against such damage – and theft – lacks economy of scale too.
However, Podargus is quite correct in saying that we shouldn’t generalise our arguments from one example. My own background knowledge arose from the days of “Small Is Beautiful “, when the alternative community believed that we could sustain a virtuous life off the renewables behind a closed farm gate. It turned out that only the big-world rich can afford to buy and sustain illusions of such genteel poverty.
Yes,Roger,there is a subset of suppliers of just about everything who will charge what the market will bear – buyer beware.
I have no experience with wind chargers so I can’t comment on their maintenance requirements.However,my solar panels get a tub with a hose brush about once per month when I hose the eucalyptus leaves off the roof and out of the gutters. It’s only the usual ubiquitous dust on the panels.If gypsum is fouling this blokes panels I hate to think where he lives.
Lightning conductors are inexpensive to install in a stand alone operation as there shouldn’t be any overhead wires as in a grid setup.
If the size of the battery bank is kept to sensible proportions by conservative use of electricity this would be the major replacement item.Probably no more frequently than 5 or 6 years.
All still a hell of a lot cheaper than running a diesel gen set up to 16 hours/day,every day.Even with the oil price at around $80/barrel and the high Australian dollar you are still looking at paying close to $2.00/litre or more out in MAMBA country. And the only place the price of oil is going is up.
The cost of bringing in diesel or fuel oil to a remote location diesel/fuel oil generator can be over 1 dollar per kWh of electricity. So solar is a good idea there. But the market for this is small – much smaller in fact than even today’s solar market (driven almost completely by hefty subsidies in Germany and California). So that’s bad in terms of the learning curve effect. As I’ve argued before solar is a very marginal energy source, and where it is not marginal it is in small market niches. Not much to do with global CO2 emissions problems at all.
By the way, according to this article:
http://www.renewableenergyworld.com/rea/news/article/2011/05/csp-targeting-grid-parity-in-spain
Spain has a CSP feed in rate of 28 eurocents per kWh. So the levelised costs of typical CSP projects isn’t much less than that (a little bit to allow higher profits for investors, but not like 50% lower that EL suggests!).
Podargus: ‘While I believe that nuclear is the only viable solution for clean base load power I think that solar,in particular, has a great future in areas where grid connection is not practical.’
Isn’t this really basically irrelevant? Developed or developing countries use a lot of energy. In these countries, people live in cities (more so as the country develops and energy use climbs). So, most energy use is or will be ‘on the grid’. If we are serious about CO2, that is what matters. Whether a few people in the outback get their power from diesel or solar may be important to them. But, they don’t really count in the big picture.
I agree. Connecting people to grids is one of the most important steps forward in welfare and general intellectual, human and economic development. It’s not just homes that we must empower – it is factories, hospitals, schools. The electricity we use in our homes is typically only 20-30 percent of the total! And that other 70-80% is mostly centralized, ie on the grid and also slightly over half of the world population live in cities as Steve points out. Urbanisation is increasing. Over 70 percent could live in cities before 2050, if current urbanisation trends continue.
Even where there are no cities, not having a grid is not something we should find acceptable. Ultimately we’ll need to connect as many to the grid as possible. This will allow people to have access to a high quality medical and educational system.
The notion of an off-grid, autonomous PV installation powering remote villages in the world is romantic, but hardly anywhere near as good as a modern grid in terms of human and economic development potential, plus it has very little to do with the energy transition (away from fossil) we have to make. It’s niche.
I know Cyril R hails from Holland but I don’t know where SteveK9 is from.
I was obviously speaking in the Australian context when I replied to Roger Clifton.Australia is a large continent.Just how large seems to escape foreigners until they come here as tourists and think they can “do” the country in a few weeks.The Eastern,South Eastern and South Western fringes are (currently) climaticly favoured and have a relatively high population density.This is where the grid is practical and nuclear power,with present reactor sizes,is the way to go.
However,the vast majority of Australia’s area is sparsely populated and in large areas is virtually uninhabited because it is extremely arid.But people live out there because they make a living there or prefer to live there,as in the case of a lot of aborigines.These people are not irrelevant.
It is not practical to extend a grid to these places,even using single wire earth return systems.Even in areas where the grid exists it can be so expensive to bring electricity onto a property that it becomes economic to install a stand alone generating system.The ever increasing cost of grid supplied electricity also has to be factored in.
There is nothing romantic about any of this.It is just hard nosed economics.
And while the big picture is important don’t lose sight of the details.
A carbon tax of $20/ton of CO2 will add about $20/MWh to the cost of coal-fired electricity. If we assume another $10/MWh in pricing, by 2020, for other coal pollutants such as heavy metals, then together these charges overcome the $30/MWh difference, given in the main post above, between first-of-a-kind nuclear and new coal plants. So right now utilities should be taking a hard look at nuclear, as should all their customers who care about rates.
As an exercise in manufacturing, and assuming a similar spend to create the plant in question; how much of the spend on a NPP (Areva, Westinghouse etc) would remain in Australia as compared to construction of a CSP/storage plant?
I’m guessing the low tech CSP plant would have a dramatically higher local contribution.
Zvyozdochka, CSP is far less productive than nuclear, so it appears to have a high employment per kWh. Of course what is really going on is that the low productivity of CSP means the workers produce fewer kWhs. CSP has fewer kWhs per worker, which is not a good thing. But if you state it as high employment per kWh it suddenly sounds great. Of course that is false advertising, analogous to you getting a house built and the contractor says he’s got the highest employment rate per house. Which is to say, he needs more workers to build your house so he’s got lazy/improductive workers and wants you to pay more money for it. Who believes this is a good thing? Most greens do, oddly enough.
The entire industrial, commercial and services sectors rely on cheap electricity and energy in general. That means the fewer workers you have in the electric sector the cheaper it gets and the more workers you get in all other sectors.
Some clarifying information on the long-term maintenance costs of cleaning solar PV panels…
An earlier assertion (of mine , challenged by Podargus ) that gypsum accumulates from desert aerosols (aka desert glaze) turns out to be quite wrong, at least, on an urban timescale. I was able to track down an engineer who has been closely associated with the solar panels around Central Australia. He said that the installation in question, circa 1994, had accumulated no such film since then, and that the occasional dew is often sufficient to clean the glass of dust, and presumably any associated salts.
Seeing as the installation was new at the time of the IEng field visit, it seems that the word “gypsum” was in my question, and not in their answer. The panels are innocent.
RC my panels sometimes get a few cm of snow. That usually slides off by mid morning, taking bird poo with it.
As a first-of-kind Australian NPP, what would be a likely scheduled outage factor? NERC (http://www.nerc.com/page.php?cid=4|43|47) have all US nuclear reactor types operating at 7.5% during 2006-2010.
This is the average across the fleet and presumably includes reactors out of service for refuelling operations, upgrades etc.
Without local operating experience would it be reasonable to expect a slightly higher figure initially? Historic US data seems to show a slowish decline to the 7.5% figure and then remaining pretty steady over the last 7-10 years.
@ Cyril R
If Australia was to purchase an NPP from Areva I’m sure there would be a component of jobs created in Australia during construction, but I’d expect a large part of the payment stream would be imported product. The local contribution would likely be plant surrounds/buildings not the reactor and advanced support systems themselves.
Would kWh per worker be a useful metric??
Scheduled Outage Factor = % (Scheduled Outage Hrs / Period Hrs)
Period Hours = Number of hours unit active
Schedule Outage Hours = Hours planned plus Maint plus Maint extension hours